1. Introduction
Klebsiella pneumoniae is recognized as an important Gram-negative opportunistic pathogen that causes community- and hospital-acquired infections globally [
1].
It can cause ventilator-associated pneumonia among patients in intensive care units (ICUs), bloodstream infections and urinary tract infections [
2,
3,
4]. The emergence of multidrug-resistant (MDR)
K. pneumoniae has become a major public health problem causing a significant increase of morbidity and mortality worldwide [
5]. The spread of carbapenem-resistant
K. pneumoniae (CRKP) has generally been considered an increasingly serious issue for clinical practice due to the limitation of therapeutic options [
6,
7,
8]. Greece is endemic for CRKP [
9,
10,
11,
12,
13]; data from the European Antimicrobial Resistance Surveillance Network committee for the year 2020 indicate that the prevalence of CRKP in Greece was 66.3%, the highest among the European countries [
14].
The rapid dissemination of CRKP is closely related to the antimicrobial resistance genes carried by plasmids and transferable genetic elements [
15,
16,
17,
18]. CRKP strains accumulate antimicrobial resistance genes due to inadequate implementation of infection control measures in healthcare settings and the irrational use of antimicrobials resulting in the emergence of MDR, extremely drug-resistant and pandrug-resistant phenotypes [
19]. The pathogenic potential of these strains is determined by virulence factors, such as capsular polysaccharide synthesis, fimbriae, pili, outer membrane protein and iron acquisition systems [
20].
The application of next-generation sequencing (NGS) has become a powerful tool for obtaining whole genome sequences (WGS), resulting in genetic characterization of the strains and a better understanding of the genomic diversity providing fast and in-depth information about the bacterial pathogenicity [
21]. WGS analysis is recommended for genotyping and determining relatedness between clinical
K. pneumoniae strains, as well as for the surveillance of virulence genes [
22]. The aim of the present study was to identify the antimicrobial resistance and accessory genes of selected CRKP isolates recovered in an adult intensive care unit (ICU) and a pediatric ICU (PICU) of a tertiary hospital in Greece, to evaluate their clonal relatedness through core genome single-nucleotide polymorphism (cgSNP) analysis and to calculate the evolution dynamics of this bacterial population.
3. Results
3.1. Antimicrobial susceptibility testing
All CRKP isolates were resistant to β-lactam antibiotics (including penicillins, cephalosporins, monobactams and carbapenems). Nineteen isolates (79.2%) were resistant to at least one aminoglycoside, 23 isolates (95.8%) showed resistance to quinolones, eight isolates (33.3%) were resistant to fosfomycin and tigecycline, and 21 (87.5%) to cotrimoxazole. Four out of five isolates that carried the class A β-lactamase
blaKPC showed susceptibility to ceftazidime-avibactam (CAZ-AVI). The phenotypic resistance rates to antimicrobials of CRKP isolates are shown in the heatmap of
Figure 1.
3.2. MLST, antimicrobial resistance genes, plasmids
The CRKP isolates belonged to eight different STs: ST11 (7, 29.1%), ST39 (7, 29.1%), ST323 (3, 12.5%), ST15 (7, 29.1%), ST307 (1, 4.2%), ST30 (1, 4.2%), ST512 (1, 4.2%) and ST35 (1, 4.2%).
The resistance genes and the plasmids content per isolate are detailed in
Table 2. The prevalence of genes conferring resistance to beta-lactams was 100%, to aminoglycosides 79.2%, to quinolones 95.8%, to fosfomycin and tigecycline 33.3%, and to cotrimoxazole 87.5%. The most prevalent plasmid was IncF which is associated with the spread of several extended-spectrum β-lactamase (ESBL) genes or carbapenemases and was detected in all CRKP strains; additional plasmids were found in fifteen strains, such as Col and IncC.
3.3. Virulence factors and Efflux and Regulator Systems
The virulence factors detected included fimbrial genes, as 23/24 isolates possessed the mrk cluster (type 3 fimbriae); siderophores, as yersiniabactin genes (ybt, irp and fyuA) were present in 17 isolates, while genes encoding aerobactin siderophore (iut) were identified in five isolates (three of them carried also yersiniabactin genes). In addition, the kfu gene (Klebsiella ferric iron uptake which is a regulator of iron transport system) was detected in four isolates.
Efflux and regulator systems genes encoding the AcrAB efflux pump (
acr, mar, sox, rob, ram, sdiA
, fis and envR) or the OqxAB efflux pump (
oqx and
rar) were present in all isolates (
Table 2).
3.4. Genomic comparison among CRKP strains
Circular genome maps were generated for the four STs with more than one isolate (ST11, ST39, ST323 and ST15). It was found that isolates belonging to the same ST shared many identical regions with the reference strain. CRKP isolates C4112 and C746 of the ST11, D2452 and D6184 of the ST39, Z866 of the ST323, and B2562 of the ST15 displayed visually the highest similarity with the reference strain throughout their whole genome (
Figure 2).
3.5. CgSNP-based phylogenetic analysis
The length of the multiple core genome alignment of all CRKP isolates was 1,892,845 base pairs. The core genome length corresponds to about 36.1% of the reference genome length. Nearly 1.9 million columns in the core genome alignment (almost 98.5%) showed identical nucleotides for all isolates, meaning that 1.5% of the genome is polymorphic.
A phylogenetic tree including the cgSNP-based phylogenetic analysis of all isolates, along with the reference strain, is seen in
Figure 3.
When performing all possible pairwise analyses among ST15 isolates, an average of 17 recombined SNPs (range 5-26) were detected; the SNP diversity along the core genome was very low, that is 0-3 cgSNPs per kilobase. ST323 CRKP strains presented an average of six recombined SNPs (range 5-6), and the SNP diversity was extremely low, that is 0-2 cgSNPs per kilobase. ST39 CRKP strains displayed an average of 16 recombined SNPs (range 4-43) with the SNP diversity being low, that is 0-5 cgSNPs per kilobase. Finally, ST11 CRKP strains showed an average of 866 recombined SNPs (range 1-1652), with the SNP diversity being clearly higher, that is 0-66 cgSNPs per kilobase.
For pairs of CRKP isolates, for instance C4112 and C746 with relatively low diversity (7.5 x 10
-4) (
Figure 4G), the impact of recombination is almost directly clear in the pattern of SNP density along the genome. While the SNP density is very low along most of the genome, that is 0-2 SNPs per kilobase, there are a few segments where the SNP density is much higher (
Figure 4A) and comparable to the typical SNP density between randomly selected highly diverged CRKP isolates, that is 30-65 SNPs per kilobase (
Figure 4C). For some pairs of isolates, for example, A18940 and D2856, with higher genetic diversity (1.5 x 10
-3) (
Figure 4G), the frequency of these high-spiking recombined regions increases (
Figure 4B), until most of the genome is covered by such regions when comparing highly divergent isolates (5.5 x 10
-3), for instance A5051 and C2482 (
Figure 4G,
Figure 4C). The distribution of SNP densities was described through a majority of clonally inherited regions with mostly up to three SNPs per kilobase, and a long tail of recombined regions with up to 50 or 65 SNPs per kilobase (
Figure 4D-F).
Figure 5 highlights the distribution of the lengths of tree-compatible stretches. This distribution had a mode at n=2, stretches were normally around 7-12 consecutive SNPs, and very rarely longer than 15-20 consecutive SNPs (
Figure 5A). Similarly, tree-compatible segments were typically just a few hundreds of base pairs long (around 1000-1500 base pairs), and very rarely more than 2000 base pairs (
Figure 5B).
Figure 6 underscores the ratio C/S for random subsets of all 24 isolates. When comprising all 24 isolates, a ratio C/S=0.15 was obtained for the 5% homoplasy-corrected full alignment. For small subsets of isolates, the C/S ratio displayed significant fluctuations. For instance, for subsets of n=7 isolates, the C/S ratio presented a mean of 0.094 ± 0.046. However, as the number of isolates in the subset increased, the ratios converged to the value C/S=0.15, and for large subsets of isolates there was little variation in this ratio. Consequently, for alignments of large sets of isolates, the phylogeny should change at least approximately every seven SNPs (
Figure 6). In addition, on average, each randomly chosen position on the core genome has been overwritten at least
T=4344 times (5% homoplasies removed).
4. Discussion
In the current study, the WGSs of 24 CRKP isolates were analysed. It was found that they belonged to eight different STs, with ST11 and ST39 being the most prevalent. Ten out of 24 isolates (41.6%) carried
blaNDM-1, and two of them (8.3%) transferred concurrently
blaNDM-1 and
blaOXA-48. Six out of 10 NDM-producers (60.0%) were assigned to ST11 which is common in NDM-positive CRKP strains, and it is globally distributed, especially in Asia, and particularly in China, but also in Europe [
37,
38]. In Greece, the first outbreak by NDM-1-producing
K. pneumoniae ST11 clonal strain occurred during 2011-2012 [
38], while the first report of
K. pneumoniae ST11 clinical isolate co-producing NDM-1 and OXA-48 carbapenemases was reported in 2016 [
39]. However, three out of 10 NDM-producers (30.0%) were assigned to ST15; this has also been previously described [
40].
ST39 was also the most prevalent type encompassing seven isolates (29.1%); two of them carried
blaKPC-2, while one of them (14.3%) harboured
blaKPC-33 (a KPC-2 variant). CRKP strains belonging to ST39 have been detected only in sporadic cases so far, like ST39 carrying
blaKPC-2 [
41], or
blaVIM-1 [
42]. ST39 isolates transferring KPC-33, have been recently detected in Greece [
8]. The present study also included the recently reported four ST39 isolates carrying both
blaKPC-2 and
blaVIM-1 [
43]. Three CRKP isolates (12.5%) were assigned to ST323; sporadic cases of ST323 CRKP have been previously reported in Greece and other European countries [
44,
45]. It is of interest that one isolate, A1746/22, carried the VEB-25 gene (article submitted for publication); VEB-25 is a variant of VEB-1 and confers resistance to CAZ-AVI [
46,
47].
Twenty of the 24 isolates harboured at least two genes encoding aminoglycoside-modifying enzymes (AMEs), and six of them carried one 16S rRNA methylase gene (
armA,
rmtB). In addition, 12 of these isolates carried at least one gene of the three AME classes (acetyltransferases-
aac, phosphotransferase-
aph, nucleotransferases-
ant). A disagreement between phenotype and genotype has been detected in five isolates (20.8%) (detection of AME-encoding resistance genes but phenotypically sensitive to both aminoglycosides tested). A disagreement of approximately 10% between genotype and phenotype for aminoglycosides for VITEK 2 automated system has been reported previously [
48].
Fluoroquinolone resistance was observed in seven isolates (29.2%), which carried the plasmid-encoded
qnr gene, as previously described for CRKP isolates [
49]. The
multidrug efflux pump oqxAB gene was detected in all strains [
50], while the
aac(6’)-Ib-cr gene (which encodes an aminoglycoside acetyltransferase that modifies not only aminoglycosides but also fluoroquinolones) has been identified in 16 isolates, as previously described [
49].
For fosfomycin resistance, the gene
fosA was detected in all isolates, but 16 of them (64.0%) were phenotypically susceptible, evidenced by an increase in the MIC, as previously described [
51]. Resistance genes for cotrimoxazole (
sul or
dfrA) were found in all isolates except one. The Inc-type plasmids detected in all isolates and the Col-type plasmids detected in 15 isolates have been reported in other studies [
43,
52].
In terms of adhesive structures, almost all isolates possessed the
mrk cluster, characteristic of type 3 fimbriae, which promotes bacterial adhesion to both abiotic and biotic surfaces [
53]. Regarding siderophores, the majority of isolates carried yersiniabactin genes that are significantly associated with pathogenesis and invasive infections in humans [
54], while aerobactin (detected in five isolates) is more specific for hypervirulent
K. pneumoniae [
55]. The acquisition of a chimeric plasmid including genes for the siderophores yersiniabactin and aerobactin, grants cefiderocol activity against CRKP strains [
56].
NGS allows for more accurate analyses regarding the clonal relatedness of strains compared to conventional typing methods [
57]. Several studies have described the molecular epidemiology of CRKP isolates through NGS using a gene-by-gene comparison or SNP analysis [
58,
59].
In pairwise comparisons, isolates of the ST15, ST323 and ST39 presented an average of 17, 6 and 16 recombined SNPs, respectively, implying the clonal relatedness of these isolates in accordance with their ST classification. Similarly, Miro et al. report the clonal relatedness of OXA-48-producing ST405 CRKP strains further studied with core genome MLST, showing between 6 and 17 cgSNPs [
57]. Moreover, Onori et al. reveal the clonal relatedness of sporadic KPC-producing ST258 CRKP strains presenting an average of 20 or 27 cgSNPs [
60]. ST15 and ST39 strains display a more increased number of cgSNPs compared to ST323 strains, and this is highlighted as a slightly more increased diversity in the corresponding clades of the phylogenetic tree (
Figure 3). However, ST15 and ST39 have been isolated over a 33- and 36-month period, respectively, while ST323 have been collected over a 2.5-month period (
Table 1). This could indicate a difference in the measured paces of the molecular clock between these clades, as previously proposed [
60].
On the contrary, ST11 isolates showed an average of 866 cgSNPs, indicating the polyclonality of these isolates. This indication is further strengthened by the variability in their content of carbapenemase and antimicrobial resistance genes (
Table 2) and by their phylogenetic division into two distinct subclades (
Figure 3). The high average of cgSNPs occurred between ST11 strains belonging to these two different subclades resulting in SNP patterns (
Figure 4A). Most probably, these high SNP density patterns result from horizontal recombination events, e.g. transfer of plasmid or phage, as previously proposed for other bacterial species [
36]. These results underscore the higher discriminatory power of cgSNP analysis compared to MLST [
57].
In addition, the current study highlighted novel ways in which these cgSNPs could be used to quantify phylogenetic structures and the role of recombination in genome evolution. It was shown that there are pairs of closely related isolates, and that most of their DNA have been clonally inherited, however most of the changes derived from recombination events. When focusing on the core genome alignment, it has been shown that until the current genetic state of CRKP strains each genomic locus has been overwritten thousands of times by recombination in the history of their evolution. Sakoparnig et al. reached the same conclusions when focusing
on E. coli isolates and they disclose a similar C/S ratio [
36]. Moreover, an attempt was conducted to calculate the probability for a pair of SNPs to be consistent with a common phylogeny, as a function of their genome distance [
61]. Several other studies have also attempted to quantify recombination in bacteria and assess the relative rate with which different lineages have recombined, focusing though on different types of bacteria [
36,
61]. To the best of our knowledge, our study is the first to accomplish such a task studying CRKP strains.
One of the basic challenges among WGS-based typing methods is the definition of a cut-off value for the identification of the clonal relatedness between isolates; however this remains doubtful, as it seems to be not only species-dependent but also population-dependent [
57,
60,
62]. Thus, a threshold range should be evaluated along with clinical epidemiological data.
In conclusion, the current study provides insight into the genetic characterization of CRKP isolates circulating during a 4-year period in the ICUs of a Greek tertiary hospital. Phylogenetic analysis showed that some of them were epidemiologically related. Given that NGS has become more affordable, its use in hospital settings is extremely helpful as the obtained data could lead the infection control and prevention strategies.
Figure 1.
MICs (μg/mL) heatmap of the 24 clinical isolates of carbapenem-resistant K. pneumoniae (CRKP) isolates according to CLSI.
Figure 1.
MICs (μg/mL) heatmap of the 24 clinical isolates of carbapenem-resistant K. pneumoniae (CRKP) isolates according to CLSI.
Figure 2.
Circular genome map of (A) seven ST11 CRKP strains, (B) the seven ST39 CRKP strains, (C) the three ST323 CRKP strains and (D) the three ST15 CRKP strains. The genome maps were produced through the BLAST Ring Image Generator (BRIG) software [
27]. The genomes of the seven ST11 CRKP isolates (A10143, A7213, A9974, C4112, C746, C833 and D2856), the seven ST39 CRKP isolates (B11395, C2482, D1598, D2452, D6184, Z508 and C1909), the three ST323 CRKP strains (Z557, D1463 and Z866) and the three ST15 CRKP strains (A10037, A5051 and B2562) were mapped separately to the reference genome
K. pneumoniae NC_012731. From the inner to the external ring, the innermost ring displays the size of the genome in kbp, followed by GC content (black), GC skew (dark green and dark purple) and
K. pneumoniae NC_012731 (red). The white vertical gaps represent sequences of the
K. pneumoniae NC_012731 reference strain that are absent in the sequences of the ST11, ST39, ST323 and ST15 CRKP strains or present sequences <50% identical to the reference genome.
Figure 2.
Circular genome map of (A) seven ST11 CRKP strains, (B) the seven ST39 CRKP strains, (C) the three ST323 CRKP strains and (D) the three ST15 CRKP strains. The genome maps were produced through the BLAST Ring Image Generator (BRIG) software [
27]. The genomes of the seven ST11 CRKP isolates (A10143, A7213, A9974, C4112, C746, C833 and D2856), the seven ST39 CRKP isolates (B11395, C2482, D1598, D2452, D6184, Z508 and C1909), the three ST323 CRKP strains (Z557, D1463 and Z866) and the three ST15 CRKP strains (A10037, A5051 and B2562) were mapped separately to the reference genome
K. pneumoniae NC_012731. From the inner to the external ring, the innermost ring displays the size of the genome in kbp, followed by GC content (black), GC skew (dark green and dark purple) and
K. pneumoniae NC_012731 (red). The white vertical gaps represent sequences of the
K. pneumoniae NC_012731 reference strain that are absent in the sequences of the ST11, ST39, ST323 and ST15 CRKP strains or present sequences <50% identical to the reference genome.
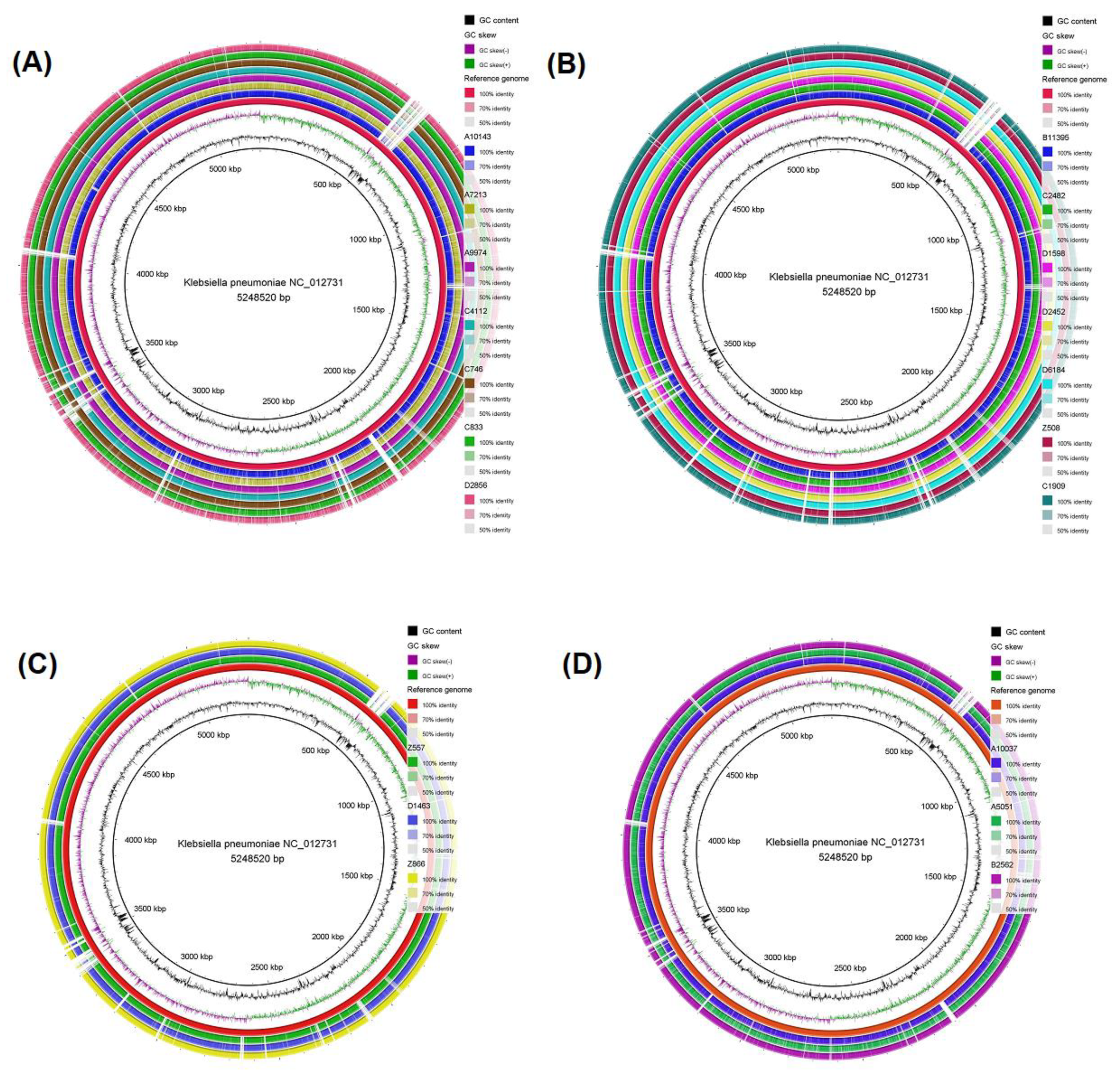
Figure 3.
The tree built on the entire core genome with PhyML. The branch color indicates the fraction of supporting versus supporting plus clashing SNPs for each branch of the core tree, which support the initial bipartition of the strains. MLST types: ST11: D2856, C4112, C833, C746, A10143, A9974, A7213, ST512: A18940, ST30: C251, ST307: Z852, ST323: Z866, D1463, Z557, ST35: A1746, ST15: B2562, A5051, A10037, ST39: D1598, D2452, C2482, Z508, C1909, B11395, D6184.
Figure 3.
The tree built on the entire core genome with PhyML. The branch color indicates the fraction of supporting versus supporting plus clashing SNPs for each branch of the core tree, which support the initial bipartition of the strains. MLST types: ST11: D2856, C4112, C833, C746, A10143, A9974, A7213, ST512: A18940, ST30: C251, ST307: Z852, ST323: Z866, D1463, Z557, ST35: A1746, ST15: B2562, A5051, A10037, ST39: D1598, D2452, C2482, Z508, C1909, B11395, D6184.
Figure 4.
Pairwise analysis of recombination between carbapenem-resistant K. pneumoniae CRKP strains. (A-C) SNP densities (SNPs per kilobase) along the core genome for three pairs of CRKP strains with various nucleotide diversities. (D-F) Corresponding histograms for the number of SNPs per kilobase (dots) together with fits of the Poisson mixture model for C4112-C746 (green), A18940-D2856 (red), and A5051-C2482 (blue) CRKP strains. The vertical axis is on a logarithmic scale. (G) For each pair of CRKP strains (dots), the fraction of the genome that was inherited clonally is shown as a function of the nucleotide divergence of the pair, shown on a logarithmic scale. The three CRKP pairs that are highlighted in panels (A-F) are depicted as the green, red, and blue dots. (H) Fraction of all SNPs that lie in recombined regions as a function of the clonally inherited fraction of the genome. (I) Cumulative distribution of the clonal fractions of CRKP pairs. (J) Cumulative distribution of the lengths of recombined segments for CRKP pairs that are in the mostly clonal regime.
Figure 4.
Pairwise analysis of recombination between carbapenem-resistant K. pneumoniae CRKP strains. (A-C) SNP densities (SNPs per kilobase) along the core genome for three pairs of CRKP strains with various nucleotide diversities. (D-F) Corresponding histograms for the number of SNPs per kilobase (dots) together with fits of the Poisson mixture model for C4112-C746 (green), A18940-D2856 (red), and A5051-C2482 (blue) CRKP strains. The vertical axis is on a logarithmic scale. (G) For each pair of CRKP strains (dots), the fraction of the genome that was inherited clonally is shown as a function of the nucleotide divergence of the pair, shown on a logarithmic scale. The three CRKP pairs that are highlighted in panels (A-F) are depicted as the green, red, and blue dots. (H) Fraction of all SNPs that lie in recombined regions as a function of the clonally inherited fraction of the genome. (I) Cumulative distribution of the clonal fractions of CRKP pairs. (J) Cumulative distribution of the lengths of recombined segments for CRKP pairs that are in the mostly clonal regime.
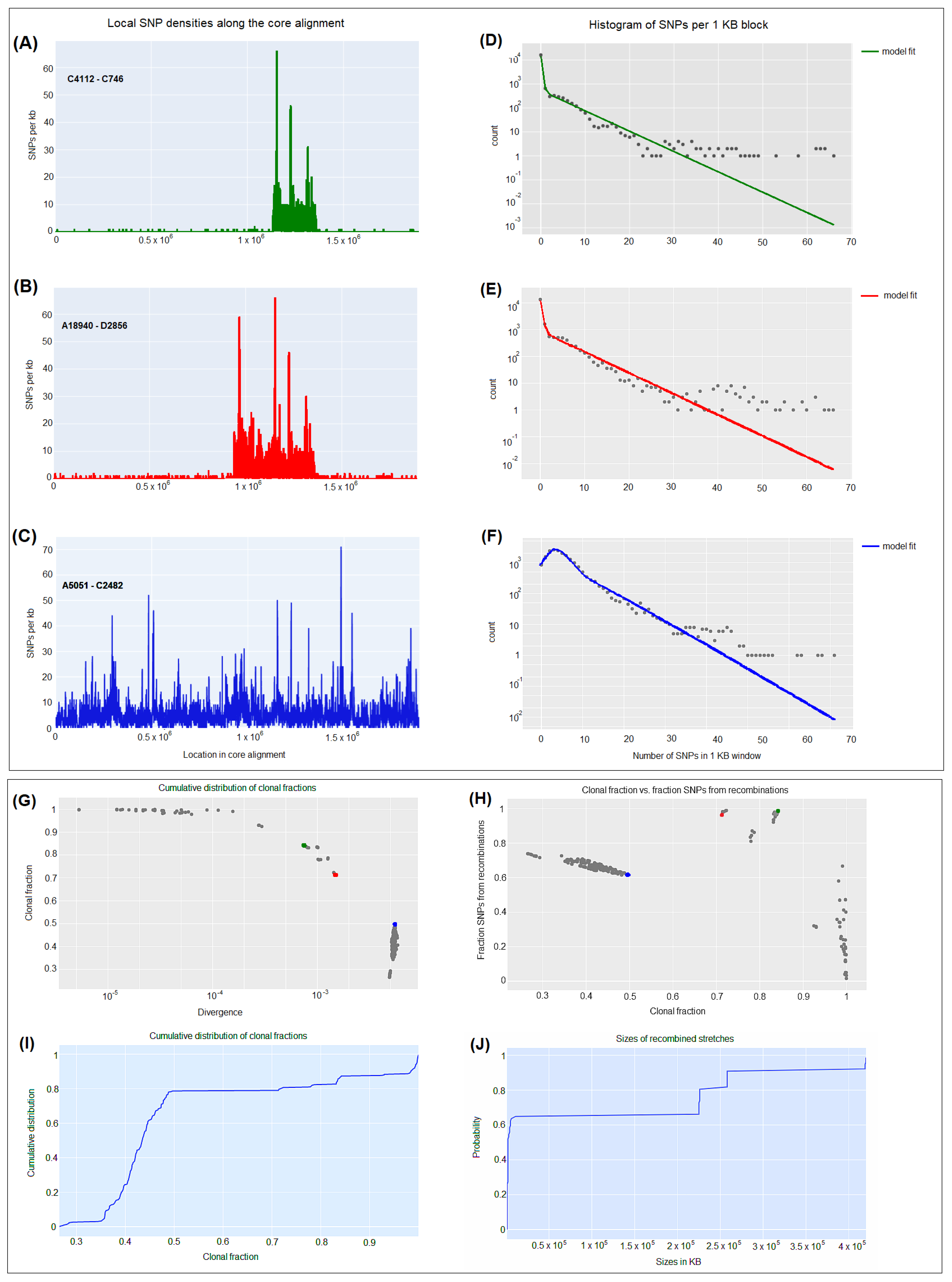
Figure 5.
SNP compatibility along the core genome alignment displays the shortness of tree-compatible segments. (A) Probability distribution of the number of consecutive SNP columns that are consistent with a common phylogeny for the core genome alignment. (B) Probability distribution of the number of consecutive nucleotides consistent with a common phylogeny for the core genome alignment.
Figure 5.
SNP compatibility along the core genome alignment displays the shortness of tree-compatible segments. (A) Probability distribution of the number of consecutive SNP columns that are consistent with a common phylogeny for the core genome alignment. (B) Probability distribution of the number of consecutive nucleotides consistent with a common phylogeny for the core genome alignment.
Figure 6.
Ratio C/S of the minimal number of phylogeny changes C to substitutions S for random subsets of carbapenem-resistant K. pneumoniae (CRKP) strains using the alignment from which 5% of potentially homoplastic positions have been removed. For CRKP strain numbers ranging from n=4 to n=24, random subsets of n strains were collected and the ratios C/S of phylogeny changes to SNPs in the alignment were calculated. The figure shows box-whisker plots that indicate, for each CRKP strain number n, means and standard deviations for C/S across subsets. The horizontal red line displays C/S=0.15.
Figure 6.
Ratio C/S of the minimal number of phylogeny changes C to substitutions S for random subsets of carbapenem-resistant K. pneumoniae (CRKP) strains using the alignment from which 5% of potentially homoplastic positions have been removed. For CRKP strain numbers ranging from n=4 to n=24, random subsets of n strains were collected and the ratios C/S of phylogeny changes to SNPs in the alignment were calculated. The figure shows box-whisker plots that indicate, for each CRKP strain number n, means and standard deviations for C/S across subsets. The horizontal red line displays C/S=0.15.
Table 1.
Clinical data of carbapenem-resistant Klebsiella pneumoniae isolates.
Table 1.
Clinical data of carbapenem-resistant Klebsiella pneumoniae isolates.
Strain |
Date of isolation |
Sex |
Age (years) |
Ward |
Biological sample |
Infection / Colonization |
C2482/18 |
9/6/2018 |
F |
8 |
PICU |
Rectal swab |
Colonization |
C4112/18 |
7/9/2018 |
F |
56 |
ICU |
Rectal swab |
Colonization |
B11395/18 |
24/10/2018 |
F |
1 |
PICU |
Urine |
Infection |
D6184/18 |
19/11/2018 |
F |
8 |
PICU |
Bronchial aspirate |
Infection |
C251/19 |
17/1/2019 |
F |
17 |
PICU |
Rectal swab |
Colonization |
Z557/19 |
30/1/2019 |
M |
57 |
ICU |
Rectal swab |
Colonization |
C746/19 |
7/2/2019 |
M |
73 |
ICU |
Rectal swab |
Colonization |
B2562/19 |
4/3/2019 |
M |
79 |
ICU |
Urine |
Infection |
Z508/19 |
20/3/2019 |
F |
60 |
ICU |
Rectal swab |
Colonization |
D1463/19 |
22/3/2019 |
M |
55 |
ICU |
Bronchial aspirate |
Infection |
D1598/19 |
1/4/2019 |
M |
40 |
ICU |
Bronchial aspirate |
Infection |
Z852/19 |
12/4/2019 |
M |
3 |
PICU |
Rectal swab |
Colonization |
Z866/19 |
15/4/2019 |
M |
49 |
ICU |
Rectal swab |
Colonization |
D2452/19 |
24/5/2019 |
M |
73 |
ICU |
Bronchial aspirate |
Infection |
C833/21 |
31/3/2021 |
F |
57 |
ICU |
Wound |
Infection |
A5051/21 |
25/4/2021 |
F |
69 |
ICU |
Blood |
Infection |
D2856/21 |
7/7/2021 |
F |
54 |
ICU |
Bronchial aspirate |
Infection |
C1909/21 |
8/7/2021 |
M |
85 |
ICU |
Wound |
Infection |
A18940/21 |
24/12/2021 |
F |
58 |
ICU |
Blood |
Infection |
A1746/22 |
25/2/2022 |
M |
0.25 |
PICU |
Blood |
Infection |
A7213/22 |
3/5/2022 |
F |
30 |
ICU |
Blood |
Infection |
A9974/22 |
16/6/2022 |
F |
67 |
ICU |
Blood |
Infection |
A10037/22 |
16/6/2022 |
F |
53 |
ICU |
Blood |
Infection |
A10143/22 |
19/6/2022 |
M |
88 |
ICU |
Blood |
Infection |
Table 2.
Genetic characteristics of the 24 carbapenem-resistant K. pneumoniae isolates of the study.
Table 2.
Genetic characteristics of the 24 carbapenem-resistant K. pneumoniae isolates of the study.