1. Introduction
Xenoliths of diamond-bearing peridotites and eclogites as well as inclusions of olivine- and silica-normative minerals in diamonds [
1] are indicative of an ultrabasic-basic evolution of the diamond-forming silicate-carbonate-(C-O-H-fluid) systems at depths of the upper mantle. A combined genesis of the diamond-bearing rocks and paragenetic inclusions in diamonds was carried out in the upper mantle diamond-forming chambers [
2]. Taken together these rocks and diamonds were transferred by kimberlitic magmas from the mantle chambers and through the cumulative centers of the Earth core into the diamond-bearing explosion tubes [
3,
4,
5,
6,
7]. The upper mantle xenoliths of native peridotites and eclogites, being as enclosing for the diamond-forming chambers, were also captured by the kimberlitic magmas [
1,
8]. It is evidently, that these rocks have been formed as a result of the ultrabasic-basic magmatism evolution in epoch of crystallization of the global magma ocean having pyrolite composition [
9].
In the absence of experimental evidence, the physico-chemical causes and mechanisms of ultrabasic-basic evolution of the upper mantle magmatic systems remain unraveled [
10,
11,
12,
13]. In this regard that is significant question of Prof. H.J. Yoder relating to the key problem [
14]: “How does crystallization of the products of partial melting of garnet peridotite are accompanied by disappearance of two main phases (olivine and orthopyroxene) and by formation of garnet and clinopyroxene only, i. e., eclogite?”.
In this connection it is required a closer look at a liquidus structure of the multicomponent system olivine-clinopyroxene/omphacite-corundum-coesite (Ol-Cpx/Omp-Crn-Coe) of the upper mantle (
Figure 1) [
15]. The composition diagram of the multicomponent system (
Figure 1a) is a complex of the elementary tetrahedrons-simplexes, two of which are ultrabasic – peridotite/pyroxenite P
1-peritectic (A) and Ol-Crn-eclogitic E
1-eutectic (B), and three simplexes are basic – Crn-Ky-eclogitic E
2-eutectic (C), Ky-Coe-eclogitic E
3-eutectic (D) and Coe-Opx-eclogitic P
2-peritectic (E). Complex coordinate framework of the peridotite-eclogite system and diagram of its liquidus structure are projected onto the divariant section Ol,Cpx-Crn,Cpx/Omp-Coe,Omp are presented in the
Figure 1b. Each simplex is a bearer of only one invariant eutectic or peritectic point.
A boundary between the ultrabasic and basic simplexes of the upper mantle substance is the inner plane Opx-Cpx/Omp-Grt-Crn of the diagram-complex. In this case a univariant cotectic Орх, Срх, Grt, L of the simplex A (2↔15 with maximum), which connects the ultrabasic peritectic P1 with the basic peritectic P2 (simplex E), has a temperature maximum (i. e., thermal barrier) in a piercing point of the boundary plane Opx-Cpx/Omp-Grt-Crn. Another thermal barrier exists at a univariant cotectic Cpx/Omp-Grt,Crn,L of the simplex B (6↔7 with maximum) between ultrabasic eutectic E1 and basic eutectic E2 of the simplex C. It should be realized that the boundaries between simplexes represent a peculiar kind of physico-chemical barriers, considering that evolution of multicomponent system is bounded by its liquidus structure in the volume of separate simplex. The eutectic points with minimal temperatures in neighboring simplexes and temperature maximums in the piercing points are also the thermal barriers as in equilibrium so in fractional conditions.
At equilibrium conditions these thermal barriers eliminate the possibility of evolution of the melts from ultrabasic to basic compositions. In this way the peritectic point of olivine may not exist and disappearance of olivine in peritectic reaction may not be realized.
Originally the peritectic reaction of orthopyroxene is effected in the processes of ultrabaic-basic evolution of the upper mantle magmatic systems. The reaction has been experimentally studied at the multicomponent system olivine-orthopyroxene-clinopyroxene-garnet (Ol-Opx-Cpx-Grt) in its polythermal section Opx
51Ol
09Grt
40-Cpx
51Ol
09Grt
40 at 4.0 GPa and 1450°C [
7,
16]. As a result, orthopyroxene disappears at the peritectic reaction with melt and clinopyroxene is formed. Similar simplified reaction of enstatite and melt with formation of diopside was studied in experiments at 2.0 GPa, 1650°C [
17] and 3.0 GPa, 1750°C [
18]. In this case the peritectic reaction of orthopyroxene is favored to evolution of the upper mantle magmatism only in limits of the ultrabasic compositions [
15].
As to the olivine problems, a contradiction arises between mineralogical data as to probability of its disappearance by the ultrabasic-basic magma evolution, on the one hand, and physico-chemical conclusion that realization of the olivine peritectic reaction at equilibrium conditions is impossible, on the other. Nevertheless, experimental finding of the solid-state reaction of olivine and jadeite with garnet formation [
19] allows for the olivine peritectic reaction and jadeite-bearing melt with garnet formation under conditions of fractional crystallization.
After peritectic point P
1 Ol,Opx,Cpx,Grt,L (
Figure 1b) olivine persists only in compositions of the univariant cotectic Ol,Cpx,Grt,L (3→4 without maximum) that is directed to the eutectic point E
1 of the adjacent ultrabasic simplex. Under conditions of fractional crystallization figurative point of the system composition is liable to move within ultrabasic compositions along the univariant cotectic Ol,Cpx,Grt,L to the eutectic point E
1 if jadeite component in the system is absent or its concentration is restricted.
Meanwhile the content of jadeite component in residual melts and respectively in general composition of the system after fractional removal of clinopyroxene has become the essential factor of the magmatic evolution. With increase of concentration of jadeite component in the system its content is first growing in clinopyroxene and then in omphacite. As a result the portion 3 of the univariant cotectic Ol,Cpx,Grt,L is gradually displaced in the direction of the boundary plane Opx-Cpx/Omp-Grt-Crn (
Figure 1b). With increasing concentration of the jadeite component it has produced a boundary system Jd-Di with completely miscible components. As a result the new voluminous frontier simplex Ol-Jd-Di-Grt is formed. This simplex is building into the boundary space between the ultrabasic and basic compositions. Thus, the role of jadeite component in a general composition of the multicomponent magmatic system is changed with its fractional accumulation from impurity state in ultrabasic peridotites to the main and system-forming component of basic eclogites.
In natural conditions the olivine peritectic reaction has become the key mechanism of ultrabasic-basic evolution of the upper mantle magmatism. Under influence of fractional crystallization, that exists due to the Earth’s gravitational field, compositions of the residual melts and correspondingly the general composition of the magmatic system are progressively changed. This is governed by the fact that crystallized solid minerals go out from the residual melts whereas the low-melting components (among these is jadeite) accumulate in the residual melts, i.e. in general composition of the fractionated system. Such increasing of jadeite component concentration in the melts provides sequential crystallization from clinopyroxene to omphacite which are phases of the continuous solid solutions jadeite-diopside.
Experimental studies at 6 GPa of melting phase relations of the upper mantle ultrabasic-basic system Ol-Jd-Di in the polythermal section Ol-Omp (= Jd
62Di
38) (Figs 2 and 3) reveal the peritectic reaction of olivine and jadeite-bearing melt with formation of garnet [
20]. As a result, it is provided the transition to the basic univariant cotectic Omp, Grt, L with formation of bimineral eclogites. It can be seen that the liquidus structure of the system Ol–Di–Jd–(reactionary Grt) [
Figure 3] is of special interest as a peculiar “physico-chemical bridge” between the ultrabasic peridotite-pyroxenite and basic eclogite rocks of the upper mantle garnet-peridotite facies. These results revealed not only genetic relations between ultrabasic and basic rocks but also the physico-chemical mechanisms of continuous fractional magmatic evolution and petrogenesis from the olivine-bearing peridotites to the silica-saturated eclogites. These data provide an explanation for the continual petrochemical trends of the rock-forming components in clinopyroxenes/omphacites and garnets of differentiated rocks of the garnet-peridotite facies [
21]
Experimentally at 6 GPa has also been investigated the system Ol-Di-Jd-(С-О-Н) with fluid components [
22] [n the polythermal section Оl
95(C-O-H)
05 –Omp
95(C-O-H)
05 (Figs. 4 and 5). It was found that the super-critical С-О-Н-fluid (with content of 5.0 wt. %) has an effect on composition and temperature of the peritectic reaction of olivine and jadeite-bearing melt with formation of garnet. The closing stages of fractional ultrabasic-basic evolution of the upper mantle magmatic melts are under control of the reaction. By experimental data the olivine peritectic reaction retains its prime significance since components of the (C-O-H)-fluid have no radical changing into melting phase relations of the ultrabasic-basic system olivine-jadeite-diopside-(reactionary Grt)-(C-O-H-fluid) at 6 GPa. Along with this it is found the metasomatic CO
2-carbonization of silicates and dissolving of H
2O-fluid in the completely mixing silicate-carbonate melts. With the participation of the (C-O-H)-fluid it has seen the topological changes in parameters of the liquidus structure, like temperature depressions for liquidus and solidus boundaries respectively from 1660 to 1580°C and from 1420 to 1290°C as well as displacement of composition of the olivine peritectic reaction by as much as 10 wt. % with growing in concentration of olivine component.
Figure 4.
Diagram of melting relations at the ultrabasic-basic system Ol - Jd – Di – (C-O-H-fluid) in its polythermal section Ol95(C-O-H)05 – Omp95(C-O-H)05 at 6.0 GPa (Litvin, Kuzyura, 2021). Symbols: L, melt; Ol, olivine (= Fo80Fa20); Fo, forsterite; Fa, fayalite; Omp, omphacite (= Di38Jd62); Grt, garnet.
Figure 4.
Diagram of melting relations at the ultrabasic-basic system Ol - Jd – Di – (C-O-H-fluid) in its polythermal section Ol95(C-O-H)05 – Omp95(C-O-H)05 at 6.0 GPa (Litvin, Kuzyura, 2021). Symbols: L, melt; Ol, olivine (= Fo80Fa20); Fo, forsterite; Fa, fayalite; Omp, omphacite (= Di38Jd62); Grt, garnet.
Figure 5.
Structure of the equilibrum peritectic liquidus of the ultrabasic-basic system Ol – Jd – Di – (C-O-H-fluid) [
22]. Position of the polythermal section Ol
95(C-O-H)
05 – Omp
95(C-O-H)
05 is shown by the dotted line.
Figure 5.
Structure of the equilibrum peritectic liquidus of the ultrabasic-basic system Ol – Jd – Di – (C-O-H-fluid) [
22]. Position of the polythermal section Ol
95(C-O-H)
05 – Omp
95(C-O-H)
05 is shown by the dotted line.
Thus, under conditions of the upper mantle fractional magmatic evolution peritectic reactions of orthopyroxene and olivine with melts provide conversion of the ultrabasic peridotite mineral association into the basic eclogitic one. It is essential that xenoliths of the diamond-bearing peridotites and eclogites in the kimberlitic explosion tubes as well as inclusions of the olivine- and silica-normative minerals in diamonds are indicative for possibility of utrabasic-basic evolution of the upper mantle diamond-forming multicomponent silicate-carbonate-(C-O-H-fluid) system. However, the problem is still not studied in physico-chemical experiments.
Meanwhile, according to the mantle-carbonatite theory [
7] genesis of diamond and associated mineral phases has realized in the upper mantle diamond-forming chambers. In these conditions the parental silicate-carbonate media of diamonds and genetically associated phases were formed with participation of streams of the C-O-H-fluids reacting with the native silicate rocks of the upper mantle. The C-O-H-fluid inclusions in diamonds and minerals of the rocks-xenoliths testify to their presence in the mantle at pressures up to 10 GPa [
23]. In this case compositions of the fluids are variable from CO
2, enriched in H
2O, to H
2O, enriched in CO
2 [
24]. Genetic links between the mantle silicate and diamond-forming silicate-carbonate systems had their origin starting metasomatic reactions of CO
2 and the upper mantle silicate minerals with formation of carbonate minerals and melts. Then the same silicate minerals and graphite were dissolved in carbonate and completely dissolved silicate-carbonate melts with participation of the C-O-H-fluid. As a result, the chambers of diamond-forming silicate-carbonate-(C-O-H-fluid)-carbon melts were formed. In the processes the capability for peritectic reaction of olivine and jadeite-bearing melt with formation of garnet has been translated to silicate components of the diamond-forming system.
Carbonate components have a priority significance for compositions of the diamond-forming melts through imparting of the capability to the melts to be effective solvents of solid carbon phases and to produce eventually the solutions being saturated with carbon with respect to diamond. Silicate-carbonate melts-solutions of carbon oversaturated to diamond are generated at temperature lowering at the diamond-forming center. This provides the spontaneous crystallization of diamond. As a result, diamonds with paragenetic mineral inclusions as well as diamond-bearing peridotites and eclogites are formed [
2]. The upper mantle consolidated diamond-bearing centers has been in existence over extended geological periods.
Ultrabasic-basic melt conversion of the multicomponent diamond-forming system olivine-orthopyroxene-clinopyroxene-garnet-(Mg-Fe-Ca-Na-carbonates)-(C-O-H-fluid) at the upper-mantle PT-conditions has not been experimentally investigated.
The main objectives of this work are the following:
(1) Experimental studies at 6.0 GPa pressure of liquidus phase relations in the diamond-forming system olivine-jadeite-diopside-(Mg-Fe-Ca-Na-carbonates)-(C-O-H-fluid) with 7.5 wt. % of the fluid. Therewith it will be established the possibility for realization of peritectic reaction of olivine and jadeite-bearing melt with formation of garnet.
(2) Evaluation of influence of carbonate components and increased content of the super-critical (C-O-H)-fluid on phase relations and PT-conditions of the diamond-forming system.
(3) Substantiation of the physico-chemical scenario for fractional ultrabasic-basic evolution of the diamond-forming silicate-carbonate-(C-O-H-fluid) melts at formation of the diamond-bearing rocks and diamonds with paragenetic inclusions of the ultrabsic and basic minerals.
3. Experimental studies
Melting phase relations of the diamond-forming system olivine (Ol) – jadeite (Jd) – diopsite (Di) - MgCO3 - FeCO3 - CaCO3 - Na2CO3 - (C-O-H-fluid) have been experimentally studied at 6.0 GPa in the polythermal section Оl74Carb*18.5(С-О-Н)7.5 - Omp74Carb*18.5(С-О-Н)7.5 where Ol = Fo80Fa20, Omp = Jd62Di38, Carb* = (MgCO3)25(FeCO3)25(CaCO3)25(Na2CO3)25. Content of the starting (C-O-H)-fluid is 7.5 wt. %. Choosing of the section permits to trace a changing of the system compositions from ultrabasic olivine-bearing to basic silica-saturated. Moreover, that is made possible to estimate going from clinopyroxene compositions, enriched with diopside component, to omphacites, enriched with jadeite component.
The diagram of melting phase relations for the system under experimental study is constructed (
Figure 8) using the electron-microscopic (
Figure 9) and analytical data (Table). This permits to determine the liquidus structure of the diamond-forming system and composition of the invariant point for the olivine peritectic reaction as the key physico-chemical mechanism of fractional ultrabasic-basic evolution of the diamond-forming silicate-carbonate melts.
Intense processes of carbonatization of the silicate components of minerals and melts of the diamond-forming system are proceeded at the action of CO
2-components of the supercritical C-O-H-fluid. This is evidenced by the numerous small-sized quenched grains of carbonates Na, Mg, Fe and Ca amongst clinopyroxenes and omphacites as well as inclusions in garnets. The newly formed carbonates add themselves to the carbonate constituent of the diamond-forming system. It is known the reactions of CO
2-fluid with olivine and another rock-forming mineral of the upper mantle with formation of Mg-carbonate [
30]. The composition of the supercritical C-O-H-fluid becomes enriched with the H
2O-component after CO
2 binding in carbonate formation.
Compositions of experimental phases of olivine are variable at the average value of (Mg
2SiO
4)
58(Fe
2SiO
4)
42. The content of jadeite component NaAlSi
2O
6 in the clinopyroxene-omphacite solid solutions is changeable within 10–67 wt.%, and concentration of pyrope component in the grossular-pyrope garnets corresponds to 9.7–46.0 wt. %. Garnets are the products of the peritectic reaction of olivine and jadeite-bearing silicate-carbonate melts with dissolved mainly H
2O-fluid. In conditions of spontaneous crystallizing the garnet single crystals may be as great as 200 µm (
Figure 9, c,e,f).
In the polythermal section Оl
74Carb*
18.5(С-О-Н)
7.5-Omp
74Carb*
18.5(С-О-Н)
7.5 under study at 6.0 GPa it is revealed the peritectic reaction of olivine (P) at 1020°C (
Figure 8). It is necessary to turn attention to that the peritectic at phase diagram of the multicomponent system is represented as the quasi-invariant “point” that is the short univariant line disposed in parallel to the temperature coordinate and along the direction the peritectic reaction is carried out. Respectively, the mating univariant cotectics, - ultrabasic Ol,Grt,Cpx/Omp,L and basic Grt,Omp,L ones, - will look like narrow divariant fields. The topological peculiarities are in agreement with the Rhines phase rule for multicomponent systems [
27].
On a basis of experimental data, the diagram of liquidus structure of the diamond-forming system Ol
74Carb*
18.5(C-O-H)
7.5-Jd
74Carb*
18.5(C-O-H)
7.5-Di
74Carb*
18.5(C-O-H)
7.5 is constructed (
Figure 10). The auxiliary thin line over the diagram is demonstrating the relative position of the polythermal section that was studied with experimental points the numeration of which is corresponding to the Table of experimental conditions and compositions of experimental phases.
The position of the peritectic point P of the diamond-forming system is substantially moved out to the boundary olivine-bearing composition as compared with the system olivine-jadeite-diopside-(C-O-H-fluid) of the upper mantle native substance with the content 5.0 wt.% of the C-O-H-fluid (
Figure 6) [
22]. Therewith the solidus temperature of the diamond-forming system is determined as 1000-1020 The position of the peritectic point P of the diamond-forming system is substantially moved out to the boundary olivine-bearing composition as compared with the system olivine-jadeite-diopside-(C-O-H-fluid) of the upper mantle native substance with the content 5.0 wt.% of the C-O-H-fluid (
Figure 6) [
22]. Therewith the solidus temperature of the diamond-forming system is determined as 1000-1020°С that is lower by 270°С relatively the silicate system Ol
95(C-O-H)
05–Оmp
95(C-O-H)
05 of the upper mantle.
Experimental evidence for the diamond-forming silicate-carbonate system Ol–Jd–Di–Сarb*-(C-O-H-fluid) as in the case of the silicate system of the upper mantle native substance Ol–Jd–Di-(C-O-H-fluid) [
22] testify that the supercritical H
2O-fluid is liberated at the solidus crystallization of the both systems and entering a self-dependent phases into their subsolidus assemblies.
The H2O-fluid was dissolved in melt together with carbonate components in solidus conditions of the diamond-forming systems and separated into the self-dependent phase at a solidification of the silicate and carbonate phases. From geochemical point of view, the consolidation of both the diamond-forming silicate-carbonate and the upper mantle native silicate systems was accompanied by their splitting into magmatic and hydrothermal systems coexisting in the open conditions.
According to the preliminary data, an evidence of the hydrothermal activity is observed in the subsolidus phase assembly of the diamond-forming system in the form of embryonic geode-similar structures being filled with silicate and carbonate phases (
Figure 11, a). It was also established the reaction of the H
2O-fluid and carbonates with formation of the water-bearing carbonate mineral nesquehonite MgCO
3·3H
2O that presents as a self-dependent mineral and as paragenetic inclusion in diamond (
Figure 11, b).
4. Discussion
An investigation of the liquidus structure of the multicomponent diamond-forming system silicate-carbonate-(C-O-H-fluid) at physico-chemical experiments at 6.0 GPa reveals the peritectic reaction of olivine and jadeite-bearing melt with formation of garnet. Therewith it is demonstrated a key role of the reaction in fractional ultrabasic-basic evolution of the parental melts for diamond and genetically associated minerals. As a result, genesis of the diamond-bearing peridotites and eclogites, which xenoliths have universally revealed themselves within the kimberlitic deposits of diamond, as well as paragenesis of the diamonds with inclusions of ultrabasic and basic minerals have obtained a rational physico-chemical explanation.
A constructive importance of the peritectic reaction of olivine and jadeite-bearing melt with formation of garnet in fractional ultrabasic-basic evolution of the upper mantle magmatic systems and genesis of the native peridotite and eclogite rocks has demonstrated by the previous experimental studies [
20,
22]. It is probably that the physico-chemical mechanisms and processes have been carried out in the epoch of crystallization of the global magmatic ocean at the upper mantle depths.
A generalized analysis of mineralogical information, relating to the upper mantle native silicate rocks and diamond-forming silicate-carbonate matter, on a basis of the experimental data for physical-chemical mechanisms of their ultrabasic-basic evolution, offer a clearer view of genetic links of the diamond-forming systems with the upper mantle native rocks. The principal conclusion lies in the fact that at formation of the diamond-forming systems with the use of the upper mantle native peridotite rocks the capability of the native peridotites for the peritectic reaction of olivine was passed on to the silicate components of the diamond-parental medium.
Hence the diamond-forming processes are representing the combined crystallization of diamonds and associated phases with formation of the paragenetic mineral inclusions in diamonds. The inclusions have analogy to the upper mantle minerals which were recrystallizing in the completely mixing silicate-carbonate diamond-forming melts. Rock-forming minerals of the diamond-bearing rocks were formed similarly and represented themselves as recrystallized analogues of the mantle minerals.
Origin of the diamond-forming systems of the Earth’s mantle is among the principal questions for the mantle-carbonatite theory of diamond genesis [
7]. Under these conditions their compositions must be suited to the requirements of the syngenesis criterium for diamonds and their paragenetic and xenogenetic mineral phases [
30]. These requirements are propagated through as the diamond-bearing peridotites and eclogites so the syngeneic mineral inclusions in diamonds.
Formation of the chambers of the diamond-parental silicate-carbonate melts with dissolved elemental carbon in the ascending streams of the supercritical C-O-H-fluid is started from metasomatic reactions of the CO
2-fluid with the upper mantle rock-forming minerals of peridotites (olivine, orthopyroxene, clinopyroxene, garnet) and/or eclogites (omphacite, garnet) with formation of carbonate Mg-, Fe-, Ca-, Na-components of the diamond-parental melts. Therewith the components of the same mantle minerals are dissolved in the carbonate melts formed and give rise to a silicate constituent at the diamond-forming medium. Origin of the carbon source has been retraced by isotopic data from the accretion of the carbonaceous chondrites to the mantle native carbon-bearing rocks [
31,
32,
33]. As a result of the reactions the completely miscible silicate-carbonate melts have formed. The silicate-carbonate melts, just like the carbonate ones, are the effective solvents of carbon that gives formation of the diamond-forming silicate-carbonate-carbon melts-solutions. As this takes place, the silicate constituent of the melts-solutions retains the capability for the peritectic reactions of orthopyroxene and olivine. The reactions make possible the ultrabasic-basic transfer of the diamond-forming melts under condition of fractional crystallization.
Exposure of the supercritical C-O-H-fluid upon the diamond-forming silicate-carbonate systems is resulted in some mass growing of their carbonate components in consequence of CO2-carbonatization of the silicate components. Therewith the content of the supercritical H2O-fluid being dissolved in silicate-carbonate melts becomes prevailing in relation to the remaining CO2-fluid. At the solidus PT-conditions the completely mixed silicate-carbonate melts with dissolved H2O-fluid are crystallized with formation of the subsolidus silicate and carbonate minerals. At the same time components of the H2O-fluid are separated out forming their own supercritical phase that becomes the chemically active agent of hydrothermal processes. In this way it has formed the supercritical hydrothermal system that is open in respect to the enclosing silicate-carbonate subsolidus association of the diamond-forming system and capable to physico-chemical reactions with it.
It is probable that not only supercritical H2O-fluid is responsible for a depression of the solidus temperature to 1000-1020°C for the diamond-forming system but also the carbonate components as of starting composition so adding ones after CO2-carbonation of silicates.
Figure 1.
Melting phase relations in peridotite-eclogite system Ol-Cpx/Omp-Crn-Coe of the upper mantle.
Figure 1a presents complex coordinate framework of peridotite-eclogite compositions and its simplexes: (A) peridotite-pyroxenite Ol-Opx-Cpx-Grt, (B) olivine-corrundum eclogite Ol-Crn-Cpx-Grt, (C) corundum-kyanite eclogite Crn-Ky-Omp-Grt, (D) kyanite-coesite eclogite Ky-Coe-Omp-Grt, (E) coesite-orthopyroxene eclogite Coe-Opx-Omp-Grt. Plane Opx-Cpx/Omp-Crn presents boundary between ultrabasic Ol-Opx-Cpx/Omp-Crn and basic Coe/Omp-Opx-Cpx/Omp-Crn volumes.
Figure 1b presents complex liquidus structure of ultrabasic-basic system peridotite-eclogite Ol-Cpx-Crn-Coe, projected from point Cpx/Omp onto divariant section Ol,Cpx-Crn,Cpx/Omp-Coe,Omp. Peritectic points for Opx are marked as P
1 and P
2 and eutectic points - as E
1, E
2, E
3. Ultrabasic univariant cotectics: 1 – Ol,Cpx,Grt,L; 2 - Opx,Cpx,Grt,L; 3 – Ol,Cpx,Grt,L; 4 - Ol,Cpx,Grt,L; 5 – Crn,Cpx,Grt,L; 6 – Cpx,Grt,Crn, L. Basic univariant cotectics: 7 – Omp,Grt,Crn,L; 8 – Crn,Omp,Ky, L; 9 – Omp,Ky,Grt,L; 10 – Omp,Ky,Grt,L; 11 – Ky,Omp,Coe,L; 12 – Coe,Omp,Grt,L; 13 – Coe,Omp,Grt,L; 14 – Coe,Opx,Omp,L; 15 – Opx,Omp,Grt,L. Simbols: L, melt; Ol, olivine; Opx, orthopyroxene; Cpx, clinopyroxene; Omp, omphacite; Grt, garnet; Coe, coesite, Crn, corundum, Ky, kyanite.
Figure 1.
Melting phase relations in peridotite-eclogite system Ol-Cpx/Omp-Crn-Coe of the upper mantle.
Figure 1a presents complex coordinate framework of peridotite-eclogite compositions and its simplexes: (A) peridotite-pyroxenite Ol-Opx-Cpx-Grt, (B) olivine-corrundum eclogite Ol-Crn-Cpx-Grt, (C) corundum-kyanite eclogite Crn-Ky-Omp-Grt, (D) kyanite-coesite eclogite Ky-Coe-Omp-Grt, (E) coesite-orthopyroxene eclogite Coe-Opx-Omp-Grt. Plane Opx-Cpx/Omp-Crn presents boundary between ultrabasic Ol-Opx-Cpx/Omp-Crn and basic Coe/Omp-Opx-Cpx/Omp-Crn volumes.
Figure 1b presents complex liquidus structure of ultrabasic-basic system peridotite-eclogite Ol-Cpx-Crn-Coe, projected from point Cpx/Omp onto divariant section Ol,Cpx-Crn,Cpx/Omp-Coe,Omp. Peritectic points for Opx are marked as P
1 and P
2 and eutectic points - as E
1, E
2, E
3. Ultrabasic univariant cotectics: 1 – Ol,Cpx,Grt,L; 2 - Opx,Cpx,Grt,L; 3 – Ol,Cpx,Grt,L; 4 - Ol,Cpx,Grt,L; 5 – Crn,Cpx,Grt,L; 6 – Cpx,Grt,Crn, L. Basic univariant cotectics: 7 – Omp,Grt,Crn,L; 8 – Crn,Omp,Ky, L; 9 – Omp,Ky,Grt,L; 10 – Omp,Ky,Grt,L; 11 – Ky,Omp,Coe,L; 12 – Coe,Omp,Grt,L; 13 – Coe,Omp,Grt,L; 14 – Coe,Opx,Omp,L; 15 – Opx,Omp,Grt,L. Simbols: L, melt; Ol, olivine; Opx, orthopyroxene; Cpx, clinopyroxene; Omp, omphacite; Grt, garnet; Coe, coesite, Crn, corundum, Ky, kyanite.
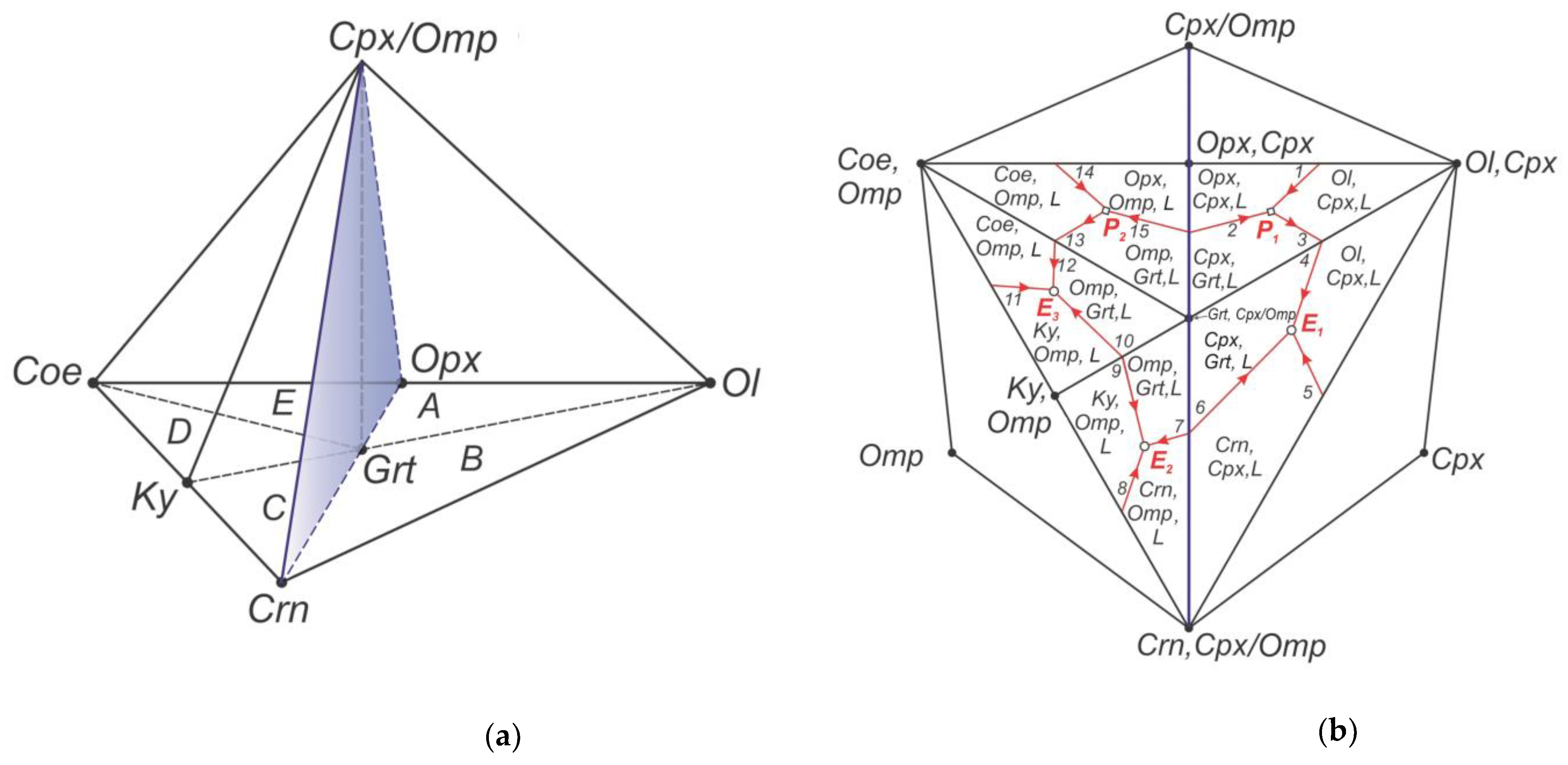
Figure 2.
Diagram of melting relations at the ultrabasic-basic system Ol-Jd-Di in its polythermal section Ol (= Fo80Fa20)–Omp (= Di38Jd62) at 6.0 GPa (Litvin et al., 2019). Symbols: L, melt; Ol, olivine; Fo, forsterite; Fa, fayalite; Omp, omphacite; Jd, jadeite; Di, diopside; Grt, garnet.
Figure 2.
Diagram of melting relations at the ultrabasic-basic system Ol-Jd-Di in its polythermal section Ol (= Fo80Fa20)–Omp (= Di38Jd62) at 6.0 GPa (Litvin et al., 2019). Symbols: L, melt; Ol, olivine; Fo, forsterite; Fa, fayalite; Omp, omphacite; Jd, jadeite; Di, diopside; Grt, garnet.
Figure 3.
Structure of the equilibrum peritectic liquidus at the ultrabasic-basic system Ol–Jd–Di (Litvin et al., 2019). Position of the polythermal section Ol-Omp is shown by the dotted line.
Figure 3.
Structure of the equilibrum peritectic liquidus at the ultrabasic-basic system Ol–Jd–Di (Litvin et al., 2019). Position of the polythermal section Ol-Omp is shown by the dotted line.
Figure 6.
Scheme of an experimental compressive cell of an anvil-with-hall (toroidal) apparatus: (1) container (lithographic limestone); (2) tubular heater (graphite); (3) thermal insulator (lithographic limestone, pyrophillite); (4) starting sample in Pt capsule; (5) capsule insulation (a mixture of MgO and hexagonal BN); the place of the thermocouple Pt70Rh30/Pt94Rh06 junction is in contract to Pt-capsule.
Figure 6.
Scheme of an experimental compressive cell of an anvil-with-hall (toroidal) apparatus: (1) container (lithographic limestone); (2) tubular heater (graphite); (3) thermal insulator (lithographic limestone, pyrophillite); (4) starting sample in Pt capsule; (5) capsule insulation (a mixture of MgO and hexagonal BN); the place of the thermocouple Pt70Rh30/Pt94Rh06 junction is in contract to Pt-capsule.
Figure 7.
Temperature-composition phase relations of the system H2O-CO2 at pressure of 6 GPa (Abramson et al., 2017) with solid phase β-H2CO3 (Wang et al., 2016).
Figure 7.
Temperature-composition phase relations of the system H2O-CO2 at pressure of 6 GPa (Abramson et al., 2017) with solid phase β-H2CO3 (Wang et al., 2016).
Figure 8.
Diagram of melting relations in the ultrabasic-basic diamond-forming system Ol-Jd–Di–Carb*–(C-O-H-fluid) in its polythermal section Ol74Carb*18.5(C-O-H)7.5–Omp74Carb*18.5(C-O-H)7.5 at 6.0 GPa. Symbols: L, melt; Ol, olivine (= Fo80Fa20); Fo, forsterite; Fa, fayalite; Omp, omphacite (= Di38Jd62); Grt, garnet; Carb*, carbonate mix (MgCO3)25(FeCO3)25(CaCO3)25(Na2CO3)25. Numbered points for experimental compositions (in the table).
Figure 8.
Diagram of melting relations in the ultrabasic-basic diamond-forming system Ol-Jd–Di–Carb*–(C-O-H-fluid) in its polythermal section Ol74Carb*18.5(C-O-H)7.5–Omp74Carb*18.5(C-O-H)7.5 at 6.0 GPa. Symbols: L, melt; Ol, olivine (= Fo80Fa20); Fo, forsterite; Fa, fayalite; Omp, omphacite (= Di38Jd62); Grt, garnet; Carb*, carbonate mix (MgCO3)25(FeCO3)25(CaCO3)25(Na2CO3)25. Numbered points for experimental compositions (in the table).
Figure 9.
Figure 9. SEM-images of the sample of the ultrabasic (a, b) and basic (c, d, e, f) compositions.
Figure 9.
Figure 9. SEM-images of the sample of the ultrabasic (a, b) and basic (c, d, e, f) compositions.
Figure 10.
Structure of the equilibrum peritectic liquidus of the ultrabasic-basic diamond-forming system Ol–Jd–Di–Carb*-(C-O-H-fluid). Position of the polythermal section Ol74Carb*18.5(C-O-H)7.5–Omp74Carb18.5(C-O-H)7.5 is shown by the dotted line. Numbered points for experimental compositions (in the table).
Figure 10.
Structure of the equilibrum peritectic liquidus of the ultrabasic-basic diamond-forming system Ol–Jd–Di–Carb*-(C-O-H-fluid). Position of the polythermal section Ol74Carb*18.5(C-O-H)7.5–Omp74Carb18.5(C-O-H)7.5 is shown by the dotted line. Numbered points for experimental compositions (in the table).
Figure 11.
SEM-images of results of hydrothermal activity at subsolidus mutter of the diamond-forming Ol–Jd–Di–Carb*-(C-O-H-fluid) system at 6.0 GPa: (a) geode-like structures; (b) inclusion of nesquehonite CaCO3·3H2O in garnet.
Figure 11.
SEM-images of results of hydrothermal activity at subsolidus mutter of the diamond-forming Ol–Jd–Di–Carb*-(C-O-H-fluid) system at 6.0 GPa: (a) geode-like structures; (b) inclusion of nesquehonite CaCO3·3H2O in garnet.
Table 1.
Table of experimental conditions and compositions of experimental phases.
Table 1.
Table of experimental conditions and compositions of experimental phases.
№ sample |
Starting composition |
Т, °С |
Duration, min
|
Chemical analyses of experimental phases, wt.% of elements oxides
|
Phase |
SiO2 |
Al2O3 |
MgO |
FeO |
CaO |
Na2O |
CO2* |
Сумма |
3291 |
[(Ol80Omp20)80 Carb20]92.5COH7.5
|
1020 |
95 |
Ol |
38.75 |
0.30 |
41.71 |
16.68 |
0.09 |
0.03 |
- |
96.80 |
Grt |
38.93 |
20.85 |
11.96 |
20.67 |
6.04 |
0.18 |
- |
98.65 |
Omp |
53.90 |
2.79 |
12.38 |
8.13 |
17.54 |
3.74 |
- |
98.47 |
L |
13.72 |
0.43 |
2.77 |
19.99 |
17.39 |
14.34 |
31.36 |
100.00 |
3292 |
[(Ol80Omp20)80 Carb20]92.5COH7.5
|
930 |
120 |
Cpx |
57.36 |
6.63 |
12.44 |
8.26 |
13.38 |
6.60 |
- |
104.67 |
Ol |
28.02 |
0.24 |
33.69 |
27.73 |
0.04 |
0.47 |
- |
90.19 |
L |
14.34 |
2.77 |
13.72 |
0.55 |
17.36 |
19.75 |
31.51 |
100.00 |
L |
10.90 |
1.19 |
31.32 |
8.27 |
3.38 |
0.84 |
44.10 |
100.00 |
3296 |
[(Ol80Omp20)80 Carb20]92.5COH7.5
|
875 |
60 |
Ol |
30.71 |
0.37 |
28.38 |
38.61 |
0.14 |
0.75 |
- |
99.28 |
Omp |
55.19 |
11.26 |
7.73 |
5.07 |
8.97 |
9.68 |
- |
97.90 |
Mg,Fe-carb |
- |
- |
41.12 |
13.66 |
6.37 |
- |
38.85 |
100.00 |
3297 |
[(Ol80Omp20)80 Carb20]92.5COH7.5
|
740 |
90 |
Ol |
30.28 |
- |
41.08 |
21.13 |
- |
0.75 |
- |
93.24 |
Omp |
55.43 |
13.90 |
7.17 |
4.69 |
7.29 |
11.26 |
- |
99.74 |
Carb |
1.72 |
- |
38.65 |
7.30 |
0.30 |
2.11 |
49.92 |
100.00 |
3323 |
[(Ol45Omp55)80 Carb20]92.5COH7.5
|
1100 |
90 |
Grt |
40.51 |
22.08 |
12.16 |
11.05 |
11.28 |
3.03 |
- |
100.11 |
L |
3.61 |
0.14 |
1.63 |
0.66 |
1.02 |
22.98 |
69.96 |
100.00 |
3325 |
[(Ol40Omph60)80Carb20]92.5COH7.5
|
1130 |
90 |
Grt |
40.25 |
21.02 |
9.18 |
12.28 |
17.14 |
0.2 |
- |
100.07 |
L |
42.64 |
9.21 |
8.86 |
5.47 |
13.31 |
3.47 |
17.04 |
100.00 |
3326 |
[(Ol40Omph60)80Carb20]92.5COH7.5
|
950 |
90 |
Grt |
40.44 |
21.21 |
9.74 |
12.24 |
15.91 |
0.11 |
- |
99.65 |
L |
23.38 |
0.04 |
0.04 |
0.11 |
3.63 |
34.89 |
37.91 |
100.00 |
Omp |
56.22 |
9.22 |
11.48 |
0.79 |
16.21 |
5.04 |
- |
98.96 |
Carb |
9.13 |
0.71 |
1.14 |
0.53 |
2.40 |
38.36 |
47.73 |
100.00 |
3322 |
[(Ol30Omp70)80 Carb20]92.5COH7.5
|
1100 |
90 |
Grt |
40.83 |
21.24 |
6.02 |
13.6 |
17.71 |
0.14 |
- |
99.54 |
L |
44.28 |
6.81 |
7.77 |
3.08 |
10.71 |
5.49 |
21.86 |
100.00 |
3309 |
[(Ol30Omp70)80 Carb20]92.5COH7.5
|
950 |
90 |
Grt |
37.11 |
16.50 |
19.11 |
3.83 |
21.39 |
0.43 |
- |
98.37 |
Omp |
51.14 |
1.97 |
14.41 |
6.30 |
20.38 |
4.91 |
- |
99.11 |
Ca,Na-carb |
0.03 |
0.11 |
0.77 |
1.53 |
15.88 |
21.13 |
60.55 |
100.00 |
Na-carb |
2.93 |
0.36 |
6.85 |
5.90 |
2.40 |
24.60 |
56.96 |
100.00 |
Carb |
4.50 |
0.79 |
14.78 |
14.99 |
10.76 |
12.58 |
41.60 |
100.00 |
Carb |
1.28 |
1.13 |
28.04 |
22.22 |
2.35 |
0.64 |
44.34 |
100.00 |
L |
28.63 |
0.85 |
11.11 |
6.59 |
12.62 |
7.10 |
33.10 |
100.00 |
3298 |
[(Ol30Omp70)80 Carb20]92.5COH7.5
|
710 |
90 |
Omp |
57.89 |
17.44 |
3.65 |
2.84 |
4.16 |
13.08 |
- |
99.06 |
Arg |
0.18 |
0.08 |
0.22 |
0.00 |
55.08 |
0.37 |
44.07 |
100.00 |
Mgs |
2.10 |
0.69 |
38.44 |
5.80 |
1.64 |
0.96 |
50.37 |
100.00 |
3310 |
[(Ol20Omp80)80 Carb20]92.5COH7,5
|
1060 |
60 |
L |
25.06 |
6.18 |
17.26 |
2.42 |
6.63 |
17.94 |
24.51 |
100.00 |
3312 |
[(Ol20Omp80)80 Carb20]92.5COH7.5
|
790 |
90 |
Omp |
48.85 |
1.55 |
12.35 |
6.18 |
19.70 |
7.87 |
- |
96.50 |
Carb |
- |
- |
0.66 |
0.89 |
49.91 |
4.87 |
43.67 |
100.00 |
|
*CO2 - counted |
|
|
|
|
|
|
|
|
|
|
|