1. Introduction
Endothelial dysfunction (ED) is a marker of vascular damage and a precursor of cardiovascular diseases such as hypertension and renal failure, leading causes of death worldwide [
1]. Angiotensin II (Ang-II) is the main effector molecule of the renin-angiotensin system. It activates several signaling pathways that regulate vascular biology and, when altered, it is a major contributor to the development of vascular diseases such as endothelial dysfunction [
2]. ED is characterized by oxidative stress, inflammation, vascular remodeling, vasoconstriction, and a prothrombotic state [
3].
The pathological basis of ED is an imbalance in the synthesis, release, or effects of factors synthesized by endothelial cells [
4], such as nitric oxide (NO), a key regulator of the cardiovascular system [
5] whose lack impairs the ability of the endothelium to modulate the physiological functions of the vascular bed [
4]. The main source of NO production in endothelium is the enzyme endothelial nitric oxide synthase (eNOS), whose activation depends on protein kinase B (Akt) [
6].
In ED, the Akt/eNOS/NO signaling pathway is altered either by the effect of Ang-II, reactive oxygen species (ROS), or inflammatory mediators (IL-6, TNF-α, protein C reactive), among other stimuli [
7,
8]. The failure of NO to diffuse to the vascular smooth muscle cells (VSMCs) has two main effects: 1) actomyosin relaxation is prevented, thus promoting the maintenance of a vasoconstricted state, and 2) VSMC proliferation is induced, causing the vessel walls to thicken (remodel) [
8,
9].
On the other hand, stimuli that promote ED, including ROS, Ang-II, oxLDL, and advanced glycosylation end products, also activate NF-κB, which induces the synthesis of proinflammatory cytokines (IL-1b, IL -6, TNF-α), chemokines (MCP-1), and adhesion molecules (VCAM-1, ICAM-1, E-selectin). This proinflammatory environment promotes the adhesion, transmigration, and activation of inflammatory cells (neutrophils, monocytes, T lymphocytes), leading to an overexpression of cytokines, chemokines, growth factors and ROS, which further contribute to the progression of inflammation and damage [
4] to target organs such as kidney and liver [
10].
Previous studies have reported that
Cucumis sativus has antioxidant and anti-inflammatory activities [11-13]. In our research group, we observed that the subfractions SF1 and SF3 of the aqueous fraction of
C. sativus aerial parts showed protective effects in vitro on Ang-II-induced ED using HMEC-1 cells. The main components found in both subfractions were glycine, arginine, asparagine, lysine, and aspartic acid [
14]. This study aims to assess in vivo the ability of a combination of SF1 and SF3, labeled C4, to control hypertension, vascular remodeling, inflammation, and renal and hepatic damage induced by Ang-II administration, as well as to preliminarily elucidate the mechanism of action underlying the activity against ED in vitro.
2. Materials and Methods
2.1. Plant material and fractions preparation
Aerial parts of C. sativus, including fruits, were collected from an edible crop, free of pesticides and fertilizers, in Xochitepec, Mexico, from July to August. Plant material was identified by Biol. Margarita Avilés Flores and Macrina Fuentes Mata (Herbario del Jardín Etnobotánico del Instituto Nacional de Antropología e Historia, INAH, Cuernavaca). Voucher specimens were stored for future reference (INAH-Morelos 3001). Plant material was kept protected from light at room temperature (RT) and dried.
Dry plant material was ground in a Pulvex electric mill (Büchi R-114, Büchi Labortechnik, Flawil, Switzerland) until particles smaller than 4 mm were obtained. An exhaustive maceration process with ethanol/water (60:40 v/v) was performed at RT to obtain the hydroalcoholic extract. This extract was concentrated by reduced-pressure distillation and then lyophilized. A sample of this extract (50 g) underwent bipartition with ethyl acetate/water, obtaining the aqueous fraction. This fraction was concentrated by reduced-pressure distillation. Aqueous fractions were suspended in methanol (700 mL) for 24 h; the liquid phase was filtered and concentrated in a rotary-evaporator (Laborota 4000, Heidolph, Schwabach, Germany), yielding the subfraction SF1, which was dried by lyophilization. On the other hand, the organic phase was suspended in acetone (700 mL), yielding the subfractions SF2 (soluble phase) and SF3 (precipitate), which finally were concentrated under reduced pressure and lyophilized.
2.2. Cell culture
Human microvascular endothelial cells-1 (HMEC-1) were provided by Aida Castillo from the Department of Physiology, CINVESTAV-IPN, Mexico City. The cells were cultured in MCDB-131 medium, supplemented with 10% fetal serum bovine (FBS), L-glutamine 10 mM, 100 U/mL of penicillin-streptomycin (Invitrogen, Carlsbad, CA, USA), 10 ng/mL of endothelial growth factor, and 1 μg/mL of hydrocortisone (Sigma) at 37 °C under a CO2 atmosphere (5%). All experiments were performed using passages 3 to 8. The cells were cultured in Petri dishes to a density of 4 × 106 cells/dish in medium supplemented with Ang-II 5000 nM and either losartan (10 μmol) or C4 (10 μg/mL of each SF1 and SF3), for 24 h.
2.3. Western blot
HMEC-1 cells were washed with PBS and lysed by incubating with lysis buffer (Tris 20 mM, pH 7.4; NaCl 150 mM, ethylenediaminetetraacetic acid [EDTA] 1 mM, pH 7.4; Triton X-100 0.5%; sodium dodecyl sulfate [SDS] 0.1%, sodium deoxycholate 0.5%), using a cocktail of phosphatase and protease inhibitors (tablets, Roche) for 15 min. The lysates were scraped off the plates and centrifuged at 9000 x g for 10 min at 4°C. Proteins in the supernatant were quantified by the Bradford method. The volume of supernatant equivalent to 20 μg of protein were denatured by boiling for 5 min in sample buffer, separated by SDS-PAGE (polyacrylamide gel electrophoresis) in 10% acrylamide gels and electro-transferred to a polyvinylidene difluoride (PVDF) membrane (Merck) using transfer buffer (Tris 25 mM, pH 8.5; glycine 193 mM; methanol 20%). The membranes were blocked for 1 h in blocking buffer Tris-buffered saline containing Tween-20 [TBS-T] 0.1%) with bovine serum albumin (BSA) 2%. Thereafter, the membranes were incubated for 2 h with primary anti-Akt (1:1000), anti-phospho-Akt (1:1000), or anti-β-actin (Cell Signaling Technology, Danvers, Massachusetts, USA). After washing five times for 5 min with TBS-T, the blots were incubated with rabbit anti-mouse (Cell Signaling) diluted at 1:2000 for 2 h. Antibody binding was detected with the SuperSignal West Dura Extended Duration Substrate solution (Thermo Scientific). The bands were analyzed using the ImageJ software (National Institute of Health, Bethesda, MD, USA), using the anti-actin signal for data normalization.
2.4. Animals
Male C57BL/6J mice (8–10 weeks old) obtained from our animal facility were used. All experiments followed the guidelines of the National Institutes of Health Guide for the Care and Use of Laboratory Animals, and experimental protocols were reviewed and approved by the Ethical Committee for the Care and Use of Laboratory Animals (Permit No. 008/2016) of the FM-UAEM. The mice were housed in four groups of five animals each and kept in the animal house of the Faculty of Medicine of the Universidad Autónoma del Estado de Morelos under pathogen-free conditions, constant temperature (21–23 °C) and humidity (45–50%), with a 12-h light/dark cycle. The experiments were repeated three times. ED was established during the first 10 weeks of the experiment. Once the blood pressure (BP) of the mice increased by 15% or more from baseline values (systolic or diastolic), the animals were considered as hypertensive. All treatments were administered for the next 10 weeks of the experiment. The control group was given saline solution only, and the other three groups were treated daily with Ang-II (0.1 μg/kg) via intraperitoneal injection throughout the experiment [
10]. One treated group was left as control, receiving only Ang-II; another was treated p.o. with Losartan (10 mg/kg/day), and the last group was orally administered with C4 (19 mg/kg of SF1 y 6.7 mg/kg of SF3) diluted in water. The amount of each fraction was determined based on a previous pilot test in which the effect of 50 mg/kg of the hydroalcoholic extract, or the ethyl-acetic or aqueous fractions was evaluated in an ED murine model (data not shown) and the yield of SF1 and SF3 per gram of the aqueous fraction. At the end of the experiment, the mice were killed by CO2 inhalation and exsanguinated.
2.5. Blood pressure measurement
Systolic blood pressure (SBP) and diastolic blood pressure (DBP) were measured in mice under surgical anesthesia (Xylazine, 10 mg/kg, i.p.) using a noninvasive blood pressure system (LE5002 system, Panlab, Barcelona, Spain) by the tail-cuff method. BP was reported as the average of seven successive measurements.
2.6. Organ retrieving
After the last BP measurement, the mice were perfused with cold PBS under surgical anesthesia (sodium pentobarbital, 30 mg/kg, i.p.). Then, the blood, liver, kidneys, and aortas were obtained.
2.7. Biochemical analysis of plasma and liver
Plasma samples were obtained by centrifugation of heparinized whole blood at 1000 × g for 10 min and stored at −80 °C for further analysis. Creatinine, urea, and uric acid levels were measured with commercial assay kits (Química Clínica Aplicada, S.A., Spain), following the manufacturer’s instructions. Livers were weighed and then macerated with ice-cold PBS (1:10 w/v). The suspensions were centrifuged at 1000 × g for 8 min at 4 °C, and the supernatants were used to quantify triglycerides and glucose, using commercial assay kits (Química Clínica Aplicada, S.A.).
2.8. ELISA
Kidneys were weighed and frozen at −80 °C until used. The organs were macerated with ice-cold PBS-PMSF (0.1%) 1:5 w/v. The suspensions were centrifuged, and supernatants were recovered and frozen at −80 °C until used. Various ELISA kits were used to determine cytokine concentrations, following the manufacturer’s instructions. OptEIA Mouse IL-1β, IL-6, TNF-α and IL-10 ELISA kits were purchased from BD (Franklin Lakes, NJ, USA), whilst mouse TGF-β ELISA kit were purchased from Applied Biosystems (Foster City, CA, USA). Briefly, 96-well flat-bottomed ELISA plates were coated with the respective capture antibody and incubated overnight at 4 °C. Non-specific binding sites were blocked by incubating for 1 h at RT with PBS-1% BSA. Aqueous kidney extracts were added and incubated for 2 h at RT. Then, the plates were incubated with the corresponding detection anti-cytokine-HRP antibodies for 1 h at RT. Bound complexes were detected with the substrate tetramethylbenzidine after 30 min of incubation in the dark. The reaction was stopped with H2SO4 2N and absorbance was measured at 450 nm at 37 °C in a VERSAmax ELISA plate reader (Molecular Devices). Cytokine concentration was calculated with standard curves for each cytokine and reported as pg/mg protein.
2.9. Histopathology
Kidneys, aortas, and livers were fixed in buffered formalin (PBS, 10% formaldehyde, pH 7.0). Then, the tissues were dehydrated and embedded in paraffin. Tissue sections (5 μm) were transferred to poly-L-lysine-coated slides (Sigma), deparaffinized, and rehydrated. For histopathological studies, the slides were stained with either the hematoxylin-eosin or Masson trichrome stain. All slides were observed under an ECLIPSE 80i microscope (Nikon, Tokyo, Japan) and analyzed with the software Metamorph v.6.1. (Molecular Devices, San Jose, CA, USA).
2.10. Statistical analysis
Data are reported as mean ± standard error (SE). Data were analyzed using the software InStat (GraphPad, San Diego, CA, USA). Significant differences among conditions were determined by ANOVA and the post-hoc Tukey’s test, with a significance value of p < 0.05.
4. Discussion
Endothelial dysfunction is characterized by sustained vasoconstriction, VSMC hyperproliferation, oxidative stress, and a proinflammatory, prothrombotic status [
3]. A major cause of ED is a decreased bioavailability of NO, since this small molecule regulates various activities of the endothelium, including the control of vascular tone, platelet aggregation, endothelial permeability, and neoangiogenesis (Laccarino et al., 2004). NO is produced in endothelial cells by eNOS. In turn, this enzyme is positively regulated by the serine/threonine kinase Akt; thus, this kinase is a key control point for eNOS activation and endothelial dysfunction [
6,
19] and a clear therapeutic target. In this work, it was shown that C4 treatment induced Akt activation and NO production in dysfunctional endothelial cells (
Figure 2). This effect could be due to the presence in C4 of glycine, arginine, and aspartic acid, the main constituents of SF1 and SF3 [
14]. Glycine has been reported to increase Akt phosphorylation while decreasing the expression of its negative regulator PTEN [20-21]. Glycine also increases the expression of eNOS mRNA and the enzyme itself [22-23]. On the other hand, arginine, in addition to being the substrate of eNOS, can stimulate the phosphatidylinositol-3-kinase (PI3K)/Akt pathway, favoring eNOS activation, NO production and its vasodilator effects, thus improving endothelial function [24-26]. Finally, while aspartic acid is not directly involved in NO synthesis, this amino acid can be converted to argininosuccinate by argininosuccinate synthetase, and then to arginine by argininosuccinate lyase; therefore, increased substrate availability for eNOS favors NO production [
27]. By increasing the bioavailability of NO, C4 may offset several pathologic features of ED. Although mice continuously received Ang-II stimulation, vascular remodeling was significantly improved in our study (
Figure 3). In addition to the effects of glycine, arginine and aspartic acid on Akt activation and NO availability, this finding could also be due to increased expression of the silent information regulatory protein 1 (SIRT1) homologue by arginine [
28]. This protein acts as an activator of histone deacetylase protein-1 (AP-1), modulating neointimal layer formation and reducing the proliferation and migration of VSMCs [
29]. As a result of the mitigation of vascular remodeling, blood pressure returns to normal levels (
Figure 1).
Another relevant marker of DE is inflammation. In this work, C4 promoted a significant increase in IL-10 expression and decreased that of IL-1β. IL-10 is an anti-inflammatory cytokine that suppresses the activation of the IL-1 converting enzyme and the subsequent processing and release of mature IL-1β [
30]. This effect could be due to glycine and arginine, as glycine has been reported to increase IL-10 production [
31], whereas arginine epigenetically regulates IL-10 through hypomethylation of its gene promoter [
32]. Furthermore, pro-IL-1β production is partially mediated by NF-κB activation [
33]. Glycine and arginine have been reported to have anti-inflammatory effects by negatively regulating NF-κB activity, inhibiting the expression of IL-6, IL-1β, IL-17, TNF-α and cyclooxygenase 2, as well as macrophage infiltration [22, 25, 34-36]. Despite these anti-inflammatory effects, neither Losartan nor C4 controlled the increase of TNF-α in this work. Another signaling pathway involved in the production of TNF-α is MAPK p38 [
37]; thus, even though glycine and arginine inhibited NF-kB activation, they may not inhibit the p38 pathway [
37].
In addition to inflammation and oxidative stress, ED causes tissue damage in different organs, particularly kidney and liver [
38]. In renal arterioles, glomerular capillaries, and peritubular capillaries, ED causes impairment of renal function and contributes to the development of acute and chronic kidney diseases [
39] such as glomerulosclerosis, characterized by an increase in mesangial volume [40-41]. Ang-II-induced ROS production leads to hyperplasia and hypertrophy of various cell types and increases the expression of cytokines and adhesion molecules [
42]. These alterations promote the synthesis of extracellular matrix proteins by activating mesangial cells and interstitial and tubular fibroblasts, stimulating plasminogen activator inhibitor 1 (PAI-1), and promoting macrophage infiltration and activation, which further potentiates inflammation and fibrosis [14,42-43]. In this work, treating mice with Ang-II resulted in renal structural alterations such as the deposition of collagen fibers, infiltration of mononuclear cells (lymphocytes, macrophages, and fibroblasts), and an increase in mesangial and glomerular area (
Figure 5). C4 reversed renal damage, possibly due to the anti-inflammatory effect described above, by inhibiting the activation of the involved cells and macrophages. Indeed, activation of the glycine receptor (GlyR, an ionotropic or ligand-gated receptor) has been reported to contribute to the hyperpolarization of macrophages, hindering their activation [
44]. On the other hand, the control of fibrosis in various tissues could be due to the content of arginine. Ramprasath et al. [
45] reported that arginine increased the expression of Nrf2, a transcription factor that promotes the expression of cytoprotective genes such as phase-2 enzymes (heme oxygenase-1, glutathione-S-transferase A1, and NAD(P)H quinone oxidoreductase 1) [
46], which can offset the fibrinogenic and oxidative damage [47-49].
Using in vivo [10,50-51] and in vitro [
52] models, several authors have shown that Ang-II causes hepatic steatosis, increasing liver weight and triglyceride concentrations. However, there are few reports on the effect of this hormone on lipid synthesis [
51]. In this study, we found that Ang-II decreased the concentration of glucose in the liver, in line with the results of Wu et al. [
52] on the effect of Ang-II on HepG2 hepatocytes. Those authors demonstrated that Ang-II activates the transcription factor SREBP2, involved in de novo lipogenesis, a process that uses glucose as a raw material to produce oleic and palmitic acid [
53], which are immediately transformed into triglycerides [
54]. Previously, we observed that chronic application of Ang-II in mice induces steatosis by activating the de novo lipogenesis pathway [
51], an effect that would be evidenced by lower intracellular glucose concentrations [51, 54]. We also observed that Losartan increased triglyceride concentrations and decreased glucose levels within the hepatocyte, in agreement with the report by Schupp et al. [
55] that Losartan induced steatosis via activation of SREBP2 and PPAR. Meanwhile, C4 reduced triglyceride levels and partly restored glucose concentration, possibly due to the glycine and arginine content in C4, as these amino acids have been reported to have anti-steatosis activity in vivo [56-57].
Steatosis itself induces lipid degradation, which in turn leads to oxidative stress and ultimately cell death due to necrosis and inflammation, which together promote collagen fiber deposition [
58]. Binding of Ang-II to the AT1 receptor of Kuppfer cells and hepatocytes increases the production of proinflammatory cytokines, including chemokines such as CXCL8 [59-60], triggering an immune response by attracting more cells to the site of injury. In addition, Ang-II has been reported to activate hepatic stellate cells through ATR1, promoting the development of fibrosis by increasing the expression of collagen I by induction of the intracellular MAPK/ERK pathway. Also promotes an increase of tissue inhibitor of metalloproteases-1 (TIMP-1) in the liver, producing disequilibrium with increased collagen and protein deposition in the extracellular matrix (ECM) [61-62]. Interestingly, C4 decreased both cellular infiltrate and fibrosis in mice, possibly due to its content of glycine, which has been reported to inhibit inflammation and liver fibrosis; however, its mechanism of action is still unknown [35, 63-64].
Overall, we found that C4 promotes the Akt/eNOS pathway, improves NO production and endothelial functions, helping to regulate blood pressure, vascular remodeling, and inflammation, whilst ameliorating tissue damage. These effects support its potential use as a phytomedicine.
Author Contributions
Conceptualization, Celeste Trejo-Moreno; Formal analysis, Gladis Fragoso; Investigation, Mario Ernesto Cruz-Muñoz, Gerardo Arrellín-Rosas, Alejandro Zamilpa and Jesús Enrique Jimenez-Ferrer; Methodology, Celeste Trejo-Moreno, Zimri Alvarado-Ojeda, Marisol Méndez-Martínez, Gabriela Castro-Martínez, Alejandro Zamilpa and Juan Baez-Reyes; Resources, Gladis Fragoso; Validation, Jesús Enrique Jimenez-Ferrer and Gladis Fragoso; Writing—original draft, Celeste Trejo-Moreno and Gladis Fragoso; Writing—review & editing, Celeste Trejo-Moreno and Gladis Fragoso.
Author Contributions
C.T.-M. and G.R.-S. were responsible for experiment design, collection, analysis, and interpretation of data, and drafting the manuscript. Z. A. A-O., M.M.-M., M. E. C-M., G. C-M., G. A-R., A. Z., and J. C. B-R. helped to perform experiments. A.Z., E.J.-F., G.F. and G.R.-S. contributed to the analysis and interpretation of the results. G.F. and G.R.-S helped to write the final version of the manuscript. All authors read and approved the final manuscript.
Figure 1.
Blood pressure kinetics: (A) systolic (SBP) and (B) diastolic (DBP). Both blood pressure values were measured in all mice every 5 weeks throughout the experiment (20 weeks). At week 10, all Ang-II-treated mice showed a significant increase in pressure from baseline (week 0). Administration of either C4 or Losartan started at week 10. Losartan and C4 decreased both pressure values at week 5 of treatment, with Losartan being more effective. Data are reported as mean ± SE, and they were analyzed by ANOVA and Tukey’s post-hoc test. Different letters indicate significant differences (p < 0.05) between groups (n = 15).
Figure 1.
Blood pressure kinetics: (A) systolic (SBP) and (B) diastolic (DBP). Both blood pressure values were measured in all mice every 5 weeks throughout the experiment (20 weeks). At week 10, all Ang-II-treated mice showed a significant increase in pressure from baseline (week 0). Administration of either C4 or Losartan started at week 10. Losartan and C4 decreased both pressure values at week 5 of treatment, with Losartan being more effective. Data are reported as mean ± SE, and they were analyzed by ANOVA and Tukey’s post-hoc test. Different letters indicate significant differences (p < 0.05) between groups (n = 15).
Figure 2.
Nitrite quantification and expression of AKT and p-AKT. A) Nitrite quantification (n = 7). B) Western blot of AKT and phospho-AKT (n = 2). C–D) AKT and phospho-AKT expression. Ang-II treatment reduced NO availability, while both treatments prevented its decrease, which can be linked to AKT phosphorylation. Data are reported as mean ± SE, and they were analyzed by ANOVA and Tukey’s post-hoc test. Different letters indicate significant differences (p < 0.05) between groups.
Figure 2.
Nitrite quantification and expression of AKT and p-AKT. A) Nitrite quantification (n = 7). B) Western blot of AKT and phospho-AKT (n = 2). C–D) AKT and phospho-AKT expression. Ang-II treatment reduced NO availability, while both treatments prevented its decrease, which can be linked to AKT phosphorylation. Data are reported as mean ± SE, and they were analyzed by ANOVA and Tukey’s post-hoc test. Different letters indicate significant differences (p < 0.05) between groups.
Figure 3.
Vascular remodeling. (A–D) Photomicrographs of hepatic arteries, 40X. (E–L) Photomicrographs of aorta stained with Masson’s trichrome (4X, E–H; 100X, I–L). (M) Microvascular remodeling, determined as the media/lumen ratio in hepatic arteries (n = 10). (N) Macrovascular remodeling, determined as the media/lumen ratio in aorta (n = 5). Ang-II induced remodeling in micro- (hepatic arteries) and macrovasculature (aorta); both Losartan and C4 reversed this effect. Data are reported as mean ± SE, and they were analyzed by ANOVA and Tukey’s post-hoc test. Different letters indicate significant differences (p < 0.05) between groups. LOS: Losartan; C4: combination of subfractions SF1 and SF3; b: bile duct; pv: portal vein; h: hepatic artery. Arrows show the hepatic artery.
Figure 3.
Vascular remodeling. (A–D) Photomicrographs of hepatic arteries, 40X. (E–L) Photomicrographs of aorta stained with Masson’s trichrome (4X, E–H; 100X, I–L). (M) Microvascular remodeling, determined as the media/lumen ratio in hepatic arteries (n = 10). (N) Macrovascular remodeling, determined as the media/lumen ratio in aorta (n = 5). Ang-II induced remodeling in micro- (hepatic arteries) and macrovasculature (aorta); both Losartan and C4 reversed this effect. Data are reported as mean ± SE, and they were analyzed by ANOVA and Tukey’s post-hoc test. Different letters indicate significant differences (p < 0.05) between groups. LOS: Losartan; C4: combination of subfractions SF1 and SF3; b: bile duct; pv: portal vein; h: hepatic artery. Arrows show the hepatic artery.
Figure 4.
Concentration of pro- and anti-inflammatory cytokines in kidney. The levels of proinflammatory (IL-1β, TNF-α and IL-6) and anti-inflammatory cytokines (TGF-β and IL-10) were measured in the kidney at the end of the experiment (week 20). Data are reported as mean ± SE, and they were analyzed by ANOVA and Tukey’s post-hoc test. Different letters indicate significant differences (p < 0.05) between groups. LOS: Losartan; C4: combination of subfractions SF1 and SF3. n =10 mice per group.
Figure 4.
Concentration of pro- and anti-inflammatory cytokines in kidney. The levels of proinflammatory (IL-1β, TNF-α and IL-6) and anti-inflammatory cytokines (TGF-β and IL-10) were measured in the kidney at the end of the experiment (week 20). Data are reported as mean ± SE, and they were analyzed by ANOVA and Tukey’s post-hoc test. Different letters indicate significant differences (p < 0.05) between groups. LOS: Losartan; C4: combination of subfractions SF1 and SF3. n =10 mice per group.
Figure 5.
Kidney damage. Representative images of: A–D) perirenal fat; E–H) renal capsule; I–L) vessels; and M–P) cortical glomerulus of control and Ang-II-, Ang-II/Losartan-, and Ang-II/C4-treated mice. Analysis of glomerular damage (Q–S) and renal profile (T) (n = 10). Ang-II induces inflammation, evidenced by infiltrate mononuclear cells and fibroblasts and deposition of collagen fibers, which were stained in blue (B, F, J, N). Treatment with C4 decreases these parameters (D, H, L, P) better than Losartan (C, G, K, O). Ang-II induced glomerular hypertrophy (Q) and mesangial expansion (R), while decreasing glomerular capillarity (S). Treatment with C4 partially reversed these parameters. Ang-II-induced increased serum creatinine levels, indicative of a compromised kidney function, were reverted by C4 and Losartan (T). Photomicrographs were taken at 100X. Data are reported as mean ± SE, and they were analyzed by ANOVA and Tukey’s post-hoc test. Different letters indicate significant differences (p < 0.05) between groups. LOS: Losartan; C4: combination of subfractions SF1 and SF3; m: mononuclear cell; f: fibroblasts.
Figure 5.
Kidney damage. Representative images of: A–D) perirenal fat; E–H) renal capsule; I–L) vessels; and M–P) cortical glomerulus of control and Ang-II-, Ang-II/Losartan-, and Ang-II/C4-treated mice. Analysis of glomerular damage (Q–S) and renal profile (T) (n = 10). Ang-II induces inflammation, evidenced by infiltrate mononuclear cells and fibroblasts and deposition of collagen fibers, which were stained in blue (B, F, J, N). Treatment with C4 decreases these parameters (D, H, L, P) better than Losartan (C, G, K, O). Ang-II induced glomerular hypertrophy (Q) and mesangial expansion (R), while decreasing glomerular capillarity (S). Treatment with C4 partially reversed these parameters. Ang-II-induced increased serum creatinine levels, indicative of a compromised kidney function, were reverted by C4 and Losartan (T). Photomicrographs were taken at 100X. Data are reported as mean ± SE, and they were analyzed by ANOVA and Tukey’s post-hoc test. Different letters indicate significant differences (p < 0.05) between groups. LOS: Losartan; C4: combination of subfractions SF1 and SF3; m: mononuclear cell; f: fibroblasts.
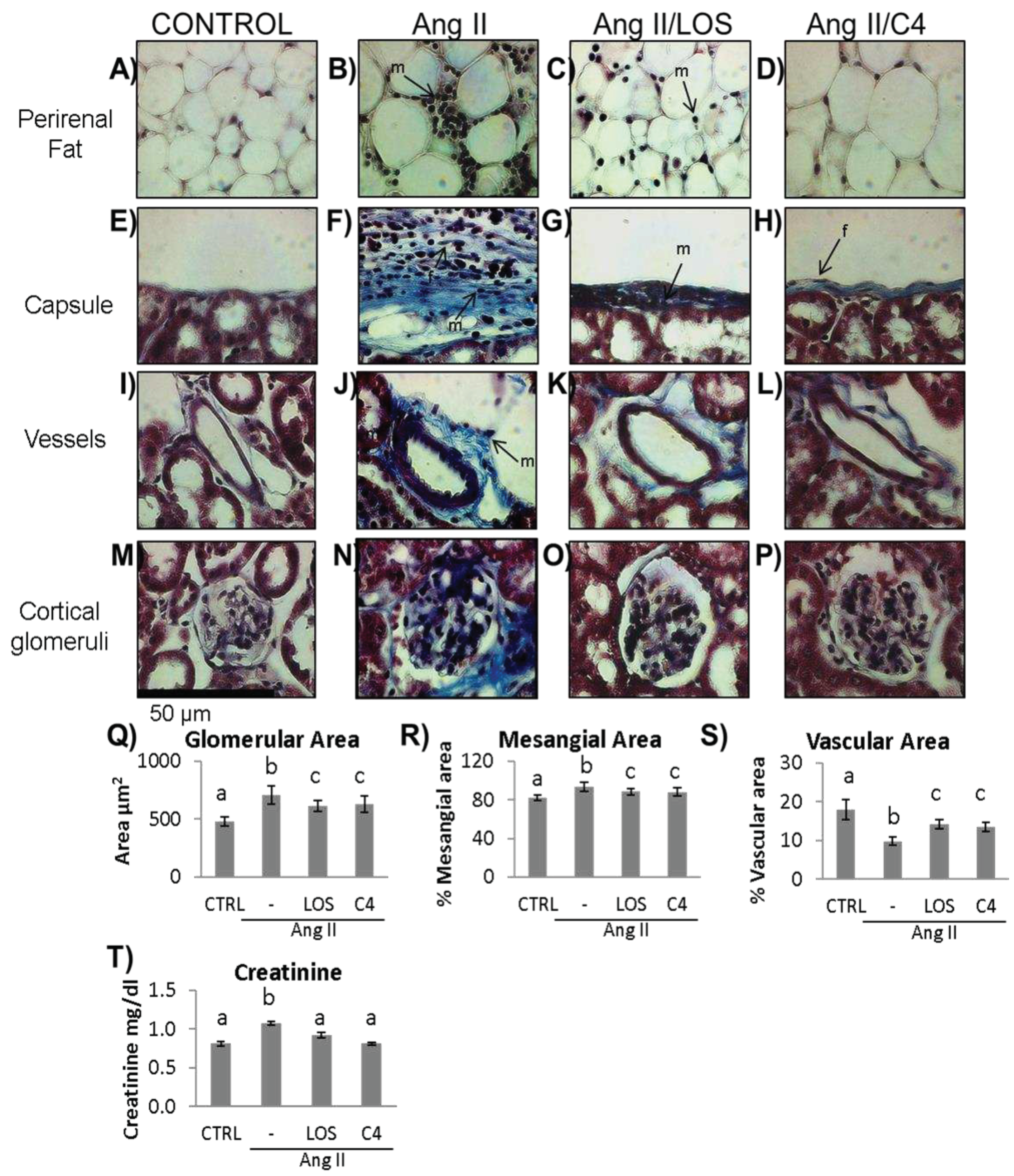
Figure 6.
Liver damage assessment by Masson’s trichrome stain. A–D) hepatic parenchyma, 4X. E–H) Glisson’s capsule, 40X; I–L) trabeculae, 40X, control, Ang-II-, Ang-II/Losartan-, and Ang-II/C4-treated mice. Ang-II-treated mice showed steatosis, mononuclear cell infiltrate, and collagen fiber deposition. Both Losartan and C4 decreased Glisson’s capsule and trabeculae thickening. Photomicrographs taken at 4X and 40X. Data are reported as mean ± SE, and they were analyzed by ANOVA and Tukey’s post-hoc test. Different letters indicate significant differences (p < 0.05) between groups (n = 5). LOS: Losartan; C4: combination of subfractions SF1 and SF3; m: inflammatory mononuclear cells; F: fibroblasts; Fb: fibrocytes.
Figure 6.
Liver damage assessment by Masson’s trichrome stain. A–D) hepatic parenchyma, 4X. E–H) Glisson’s capsule, 40X; I–L) trabeculae, 40X, control, Ang-II-, Ang-II/Losartan-, and Ang-II/C4-treated mice. Ang-II-treated mice showed steatosis, mononuclear cell infiltrate, and collagen fiber deposition. Both Losartan and C4 decreased Glisson’s capsule and trabeculae thickening. Photomicrographs taken at 4X and 40X. Data are reported as mean ± SE, and they were analyzed by ANOVA and Tukey’s post-hoc test. Different letters indicate significant differences (p < 0.05) between groups (n = 5). LOS: Losartan; C4: combination of subfractions SF1 and SF3; m: inflammatory mononuclear cells; F: fibroblasts; Fb: fibrocytes.