1. Introduction
1.1. 5G Wireless Communication
The advent of fifth-generation (5G) wireless communications has brought about the promise of ultra-fast data rates, low latency, and improved spectral efficiency by exploiting the millimeter-wave spectrum for the first time in mobile communication infrastructures (Hong et al., 2021). This technology marks a significant advancement for next-generation wireless systems, as it offers the potential for higher bandwidth and data rates (Yau et al., 2018). The Terahertz (THz) band, nestled within the expansive Radio Frequency (RF) spectrum, represents an intriguing domain spanning from 0.1 to 10 THz, characterized by its vast expanse and relative emptiness in terms of regulatory occupancy. This uncharted territory holds considerable promise for the forthcoming Sixth- Generation (6G) wireless networks, offering a rich tapestry of opportunities for innovative applications and advancements in wireless communication technology (Serghiou et al., 2022). With its relatively unexplored nature, the THz band presents a fertile ground for researchers and industry stakeholders to explore novel transmission techniques, propagation characteristics, and spectrum utilization schemes, potentially revolutionizing the landscape of wireless connectivity. Additionally, its inherent properties, such as high carrier frequencies and broad bandwidth availability, open avenues for achieving unprecedented data rates, ultra-low latency, and enhanced spectral efficiency, thereby propelling the evolution of wireless networks into realms previously deemed unattainable.
1.2. Milimeter Wave
The demand for this high-speed, high-capacity wireless communication has been steadily increasing with the proliferation of mobile broadband applications. As such, researchers and industry experts have turned their attention to the millimeter-wave (mmWave) frequency spectrum, which spans from 3-300 GHz, as a potential solution for addressing the growing needs of future wireless systems. In this literature review, we will explore the current state of research on mmWave technology and its potential implications for mobile broadband applications, including 5G and future 6G networks. Al- Al-Omary, (2020) accepted the idea and this supports the notion that mmWave technology holds promise for addressing the increasing demands on wireless networks.
While MM wave is use, there are a lot of challenges that were needed to be accomplished. The challenges of integrating millimeter-wave (mmWave) technology into 5G communication systems encompass limited propagation characteristics, susceptibility to atmospheric conditions, the need for dense small cell networks for coverage, efficient beamforming techniques to mitigate signal blockage and interference, advancements in antenna design and signal processing algorithms for optimal spectral efficiency and system capacity, as well as regulatory considerations regarding spectrum allocation and licensing.
1.3. Antenna Array
The development of millimeter-wave (mmWave) and antenna array technology has seen significant advancements in recent years, particularly in the context of 5G applications. Ghosh and Sen (2019) provided an inclusive overview of array antenna design for mmWave communications. The survey encompassed various aspects of array antenna technologies, including beamforming, polarization, and array configurations. The study shed light on the diverse design considerations and challenges associated with mmWave antenna arrays, offering valuable insights into the current state of research in this domain.
Overall, this literature review demonstrates the past advancement about mm-wave and antenna array technologies that play a critical role in addressing the unique challenges and requirements of 5G technology. These technologies enable large bandwidths, improved radiation characteristics, and flexible communication solutions, making them indispensable for the successful deployment and operation of 5G wireless networks.
2. Related Works
2.1. Broadband mm-Wave Microstrip Array Antenna
In a study by Khalily et al. (2018), they proposed for various 5G applications, a broadband millimeter-wave microstrip array antenna with better radiation properties. The research aimed to address the need for antennas capable of supporting a wide range of frequencies and applications in the mm-wave spectrum.
Analyzing the radiation and impedance characteristics of a single inset-fed patch antenna with a parasitic patch is part of the design. To reduce mutual coupling and enhance radiation pattern symmetry, the antenna array uses 16 components in an H-plane novel arrangement with an alternating out-of-phase 180° rotating sequence. With -15 dB reflection coefficients and 20 dB mutual coupling between the elements, the measured bandwidth is reported to be 24.4% (24.35 to 31.13 GHz). The proposed phased array antenna prototype shows high gains, low scan losses, narrow beamwidths.
Figure 1.
Antenna Design#1.
Figure 1.
Antenna Design#1.
The antenna design successfully addresses challenges of MM- wave frequency path loss, demonstrating small beamwidths, low scan losses, and high gains, and efficient focusing for 5G applications.
2.2. Compact Wideband Four Element Optically Transparent MIMO Antenna for mm-Wave 5G Applications
Desai et al (2020) created a transparent four-element MIMO antenna with a design of a square ring-shaped antennas formed in a common ground plane. The paper presents a solid wide-band optically transparent MIMO antenna for mm-wave 5G applications, outperforming other antennas in terms of various parameters and showing potential for use in smart devices for 5G networks.
The antenna consists of a conductive patch made from AgHT-8 (a material comprising silver layered between tin oxide layers) and a complete ground plane, with Plexiglas serving as the substrate material. The individual transparent component measures 12 mm by 10 mm and 1.85mm total thickness . The Plexiglas substrate has specific properties including a permittivity (εr) of 2.3, a low loss tangent (Tan δ) of 0.0003, and a thickness of 1.48 mm. The AgHT-8 material boasts a conductivity of 125,000 S/meter and a thickness of 0.177 mm.
Figure 2.
Antenna Design#2.
Figure 2.
Antenna Design#2.
The proposed transparent MIMO antenna system is compact and achieves dual- band operation appropriate for mm-wave 5G applications. The antenna construction also demonstrates a decent MIMO performance with satisfactory diversity parameters. The transparent Multiple Input Multiple Output (MIMO) system achieves an optical transparency of 85%, with impedance bandwidths of 12% and 28.86% at specific frequency bands. Additionally, it demonstrates gain and efficiency values ranging from 3 dBi to 75%. The diversity parameters, including MEG ratio, TARC, ECC, and MEG for a 4-element MIMO with an unpartitioned ground, adhere to recommended thresholds, indicating the potential suitability of the proposed configuration for integration into compact smart devices for mm-wave 5G networks.
2.3. Infinity Shell Shaped MIMO Antenna Array for mm-Wave 5G Applications
Yang et al (2021) prosposed an antenna design that was converted into a four-port MIMO array, measuring 30 mm by 30 mm. The MIMO setup was configured so that the radiating elements were positioned 90 degrees apart, aiming to minimize coupling effects and enhance pattern and spatial diversity. Using copper known for its stable conductivity of 5.8 × 10^7 S/m, was selected for the radiating element to ensure minimal impact on impedance matching. The design of the antenna was simulated using Computer Simulation Technology CST (2017) with a high-resolution meshing environment. The work aims to develop and investigate a unique single layer MIMO antenna design for 5G mm-wave communication in the 28 GHz frequency region.
Figure 3.
Antenna Design #3.
Figure 3.
Antenna Design #3.
The methodology involved designing the MIMO antenna Computer Simulation Technology CST (2017), fabricating it using an LPKF machine, analyzing the S-parameter response, and modeling the design in CST under a higher meshing environment.
The proposed MIMO antenna design achieved a gain of 6.1 dBi and a measured gain of 5.5 dBi, high efficiency with values of 90% and 92%, and low coupling effects with an Envelope Correlation Coefficient (ECC) of less than 0.16.
The design shows successful performance of a novel infinity-shaped MIMO antenna for 28 GHz mm-wave applications, highlighting its low mutual coupling, high efficiency, and gain for future 5G-based mm-wave communication.
2.4. A MIMO Dielectric Resonator Antenna with Improved Isolation for 5G mm-Wave Applications
The design consists of mounting two rectangles DR with a relative permittivity of 9.8 onto a Rogers 5880 substrate with specified features such as permittivity (εr) of 2.2, loss tangent (tanδ) of 0.0009, and thickness of 0.254 mm. Each DR has a microstrip-fed rectangular excitation gap located beneath for excitation purposes. Additionally, a strip of metal with length (Lp) and width (Wp) is created on the topmost layer of each DR to improve isolation between the two antenna components.
Figure 4.
Antenna Design # 4.
Figure 4.
Antenna Design # 4.
The proposed antenna achieved a simulated impedance bandwidth covering the 28 GHz band allocated for 5G applications, with a significant 12 dB improvement in isolation over the specified frequency range.
2.5. A SAR Reduced MM-Wave Beam-Steerable Array Antenna with Dual-Mode Operation for Fully Metal-Covered 5G Cellular Handsets
Bang et al. (2018) proposes a 28 GHz beam-steering array antenna designed with SAR reduction and dual-mode capabilities, tailored for use in a 5G cellular handset fully covered in metal, showcasing excellent beam-steering characteristics and providing hemispherical coverage with its beam., with calculated peak SAR values for different beam scan angles.
The proposed antenna design for a metal-covered handset made of two subarrays, with each containing 8 slot antenna elements. Subarray #1, located on the back cover, reduces SAR levels when the handset is in talk mode. Subarray #2, positioned on the upper frame, facilitates line-of-sight propagation for data mode. The slot antenna elements are strategically placed and rotated to optimize beam coverage, with dimensions and feeding locations carefully chosen for impedance matching and resonance at 28 GHz. The design employs phased array architecture, with subarray selection controlled by a microwave switching component, ensuring efficient 5G handset functionality under dual-mode operation.
Figure 5.
Antenna Design #5.
Figure 5.
Antenna Design #5.
The result of the design shows that it offers a solution for mm-wave 5G cellular handsets by providing a 28 GHz beam-steering array antenna, engineered to minimize SAR, offers dual-mode functionality through two subarrays. The design showcases strong performance in impedance matching, gain level, beam coverage, and SAR reduction, positioning it as a compelling option for integration into 5G handsets fully enclosed in metal.
2.6. Switched Folded Slot Phased Array Antenna for mm Wave 5G Mobile in Metal Bezel Design
Kim et al (2018) introduces a switched folded slot phased array antenna for mmWave 5G communication in a metal bezel mobile application, enabling beam steering in multiple planes with specific gain values and angles, supported by detailed design, simulation, and measurement discussions.
The antenna design include of two folded slot antenna arrays placed on the metal bezel of a 5-inch mobile device, with each array containing four elements. The folded slots are strategically positioned to emit radiation in either the bottom-front or bottom-rear direction of the device, enabling beam steering for different operational modes. The design incorporates a Single-Pole, Dual-Throw (SPDT) switch between the arrays to select between different radiation patterns. Near-field measurements indicate realized gains ranging from 2.8dBi to 4.2dBi, with a half-power beamwidth (HPBW) of approximately 80 degrees across all modes.
Figure 6.
Antenna Design #6.
Figure 6.
Antenna Design #6.
2.7. Multiband Millimeter Wave Antenna Array for 5G Communication
Prithu Roy et al. (2016) presented a simulated design of a millimeter wave square patch antenna 1 by 6 array on silicon and Roger RO4003 substrate for prominent multiple bands, for example: 58GHz-60GHz, 65GHz-68GHz, and 72GHz-77GHz. Designed antenna can work on 5G cellular network as well as advance device-to-device (D2D) network.
Figure 7.
Antenna Design #7.
Figure 7.
Antenna Design #7.

Because the antenna is designed with slots for inset feed, it displays various radiation bands. Six key bands have been identified from a large number of bands that can be used for 5G communication system downlink as well as D2D networks, the Internet of Things, and cellular uplink.
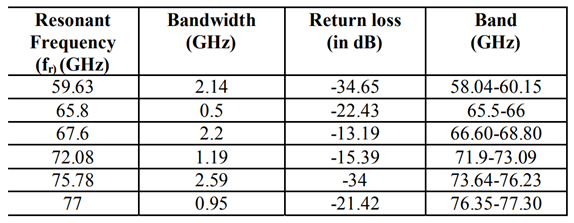
In this paper, a unique antenna array designed for 5G networks that supports cellular and device-to-device (D2D) communication is presented. With a continuous spectrum of more than 1GHz per band, the array's strong gain across many bands allows for quick data transfer. An important accomplishment is the reduction of undesired radiation with an omni-directional radiation pattern made possible by a 1 mm silicon-coated feed-line. With its tiny dimensions, conformal design, and efficient fabrication, the antenna design satisfies the criteria of the 5G system and can be mass-produced. The use of silicon substrate lowers expenses dramatically, attracting features that are affordable. Along with possible health risks from prolonged exposure to mmWave frequencies, challenges such attenuation from rain, oxygen, and shadowing are noted. To solve these challenges, research is being conducted, especially in the 60GHz and 73GHz frequencies.
2.8. 5G Millimeter Wave Antenna Array: Design and Challenges
According to research published in a paper by Jing Zhang et al. (2016), the circular antenna array is resistant to angle changes brought on by antenna movement, particularly in outdoor settings, because it maintains constant gain across a range of angles in its radiation pattern. This is in contrast to traditional antenna arrays, which frequently experience significant gain fluctuations. Along with the design issues for mmWave antenna arrays, the study also looks at methods like cooperative multi-hop relaying and distributed antenna systems to guarantee efficient mmWave communication coverage. The study also explores obstacles to mmWave cellular network implementation, including hardware development, communication security, and blocking, and suggests possible solutions to these problems.
Figure 8.
Illustration of the MMW Cellular Networks with Technology Improving Coverage.
Figure 8.
Illustration of the MMW Cellular Networks with Technology Improving Coverage.
This paper investigates the effects of various antenna array architectures on millimeter-wave (mmw) communication systems in practical outdoor settings. It draws attention to the benefits of circular antenna arrays, which exhibit reliable gain and resistance to beam misalignment. Potential fixes like multi-hop relaying and distributed antenna systems (DAS) are investigated in order to guarantee efficient coverage and connectivity in mmw systems that are prone to blockage. Furthermore, the deployment of millimeter-wavelength communications presents a number of research hurdles, such as penetration problems in forested areas, communication security issues, optimization of energy efficiency, and sophisticated hardware development. To tackle these issues, a range of approaches and possible fixes are presented, from high-density AP deployment for better energy efficiency to massive MIMO and dense relay deployment to boost penetration and security. But hardware development is still a challenge specifically for components like ADC in mmw communication systems.
2.9. Design of Millimeter Wave Antenna Arrays for 5G Cellular Applications Using FEKO
Gopinath Gampala et al(2016) discusses the concept of massive MIMO, employing antenna arrays and beamforming methods to meet the escalating demands for high data rates. They delve into various state-of-the-art technologies pivotal in setting the framework for the forthcoming 5G standards.
Figure 9.
Antenna Array design.
Figure 9.
Antenna Array design.
The antenna arrays are arranged so that the configurations of the arrays are perpendicular to one another. The result of this orthogonal orientation is polarization diversity is the outcome of spatial variety. The capacity of the mobile device to accommodate several antenna arrays furthermore provides the ability to operate in multiple configurations such as stimulating one array at a time or stimulating two arrays simultaneously
Globally, researchers are focusing on creating new technology to address the growing need for more rater of data. These innovations will also establish the framework for 5G is the next generation of mobile network specifications. An answer to the idea suggests a greater data rate requirement which is a massive MIMO. The frequency bands for mmWave suggested which the FCC has already controlled provides the opportunity to combine many antenna arrays into a compact mobile mobile phone.
2.10. Broadband Planar Antenna Array for Future 5G Communication Standards
Ullah and Tahir (2019) present a pioneering research study unveiling a cutting-edge 5G antenna array specifically designed for millimeter-wave communications. The proposed antenna array, with dimensions of 40 by 15 square mm, operates within the 23.76–42.15 GHz frequency range. A carefully crafted planar 4-way feed network has been intricately developed and fine-tuned to effectively stimulate the antenna array elements. Each individual array element features a spiral monopole encircled by vias, creating an extended ground plane in an inverted U-shaped arrangement. Additionally, hexagonal parasitic patches have been seamlessly incorporated at the rear to optimize the impedance performance of the antenna element.
Figure 10.
Structure of the proposed array antenna (a) Front side, (b) Backside.
Figure 10.
Structure of the proposed array antenna (a) Front side, (b) Backside.
The proposed antenna array achieves a peak gain of 11.5 dBi with a radiation efficiency surpassing 83% throughout its operational spectrum, showcasing a narrow beam-width as minimal as 10.1°. Utilizing standard printed circuit board (PCB) processes, both individual elements and a 1 × 4 array have been successfully fabricated. Experimental measurements closely correspond with the anticipated design goals, showcasing outstanding performance. With its cost-effectiveness, compact profile, and straightforward design, the antenna presents advantages conducive to its deployment in forthcoming 5G communication mobile networks.