1. Introduction
Following agonistic stimulation of G protein-coupled receptors (GPCRs), GPCR kinases (GRKs) phosphorylate the receptors to provide high-affinity sites for arrestins [
1,
2]. Arrestins connect the activated receptors to endocytic adaptors, such as adaptor protein (AP)-2 and clathrin [
3,
4,
5].
Both GRK2 and arrestin3 are known to undergo Mdm2-mediated ubiquitination when receptors are activated by agonists [
6,
7,
8]. Ubiquitination of GRK2 and arrestin3 could be an important cellular process that controls receptor functions, for example, the downregulation of GRK2 and arrestin3 [
7,
9].
The
Mdm2 gene, which was originally identified as an oncogene from transformed BALB/c mouse 3T3DM cells [
10], functions as a RING finger-dependent E3 ubiquitin ligase [
11,
12,
13]. Mdm2 contains a nuclear localization signal (NLS) and nuclear export signal (NES), and it constantly shuttles between the nucleus and the cytoplasm [
14,
15]. Mdm2 is present at much lower levels in the cytoplasm compared with the nucleus, and little Mdm2 is detected at the plasma membrane to regulate GPCRs [
16]. Therefore, for a cytosolic protein to be ubiquitinated by Mdm2, either the cytosolic protein needs to move into the nucleus or Mdm2 must move out of the nucleus.
A previous study indicated that the increase in the Mdm2-mediated ubiquitination of arrestin3 when GPCRs are activated by agonists occurs in the nucleus, and the pivotal factor governing this process is the agonist-induced translocation of arrestin3 into the nucleus [
8]. As expected from its main distribution in the cytosol, GRK2 does not possess an NLS. Consequently, for GRK2 to undergo ubiquitination by Mdm2, Mdm2 needs to translocate to the cytoplasm. According to our initial experiments, it was noted that upon activation of GPCRs, Mdm2 translocated from the nucleus to the cytosol. However, further studies are required to elucidate the mechanism underlying this phenomenon.
Both GRK2 and arrestin3 are ubiquitinated by Mdm2, a process enhanced by GPCR agonist stimulation. Despite some controversies, our experimental results suggest that Mdm2-mediated ubiquitination of arrestin3 increases in the nucleus, while GRK2 ubiquitination increases in the cytosolic region. Moreover, their ubiquitination processes are closely related. In this study, we investigated the molecular mechanisms involved in the mutual regulation of GRK2 and arrestin3 ubiquitination across different subcellular domains.
2. Results
2.1. Comparison of the Trafficking Patterns of Arrestin3 and GRK2 between the Nucleus and Cytosol
Mdm2, an E3 ubiquitin ligase, is located in the nucleus in its basal state, but it dynamically translocates to the cytoplasm when certain external stimuli, such as agonists of G protein-coupled receptors (GPCRs), are applied, resulting in the ubiquitination of cytosolic proteins [
9,
17]. Thus, agonist-induced nuclear export of Mdm2 can be the critical cellular event in the Mdm2-mediated ubiquitination of cytosolic proteins.
Arrestin3, not GRK2, harbors the NLS, suggesting distinct interaction mechanisms with Mdm2. In contrast to the interaction between arrestin3 and Mdm2 in the nucleus after agonistic stimulation of GPCRs [
8], the interaction between GRK2 and Mdm2 occurred in the cytoplasm (
Figure 1A). These findings were further corroborated by immunocytochemistry. As depicted in
Figure 1B and
Figure 1C, both arrestin3 and GRK2 were primarily localized in the cytosol under basal conditions. In cells exposed to leptomycin B (LMB), an inhibitor of nuclear export, a fraction of arrestin3 was detected within the nucleus, whereas GRK2 remained localized in the cytoplasm (compare vehicle- and LMB-treated cells).
2.2. Arrestin, Gβγ, Clathrin, and Receptor Phosphorylation are Involved in the Mdm2-Mediated Ubiquitination of GRK2
As a first step to understanding the molecular mechanisms involved in the nuclear export of Mdm2, the cellular components involved in the GRK2 ubiquitination were searched. As reported previously [
7], knockdown of arrestin3 abolished GRK2 ubiquitination (
Figure 2A). Arrestin3 knockdown effects were restored with the co-expression of WT-arrestin3, not K11/12R-arrestin3 which fails to undergo Mdm2-mediated ubiquitination (
Figure 2B). These results indicate that arrestin3 is necessary for GRK2 ubiquitination and further imply that the ubiquitination of arrestin3 is also essential.
Based on the results indicating that the ubiquitination of arrestin3 is implicated in the ubiquitination of GRK2 (
Figure 2B), we hypothesized that the factors involved in the ubiquitination of arrestin3, for example, Gβγ and clathrin [
8], would likewise play a role in the ubiquitination of GRK2.
Figure 1.
Roles of Mdm2-mediated GRK2 ubiquitination that occurs in the cytoplasm. (A) HEK-293 cells expressing β2AR or D2R (1.9–2.1 pmol/mg protein) were transfected with GRK2 and FLAG-Mdm2. Subcellular fractionation was conducted into the nuclear fraction (NF) and cytoplasmic fraction (CF), and each fraction was immunoprecipitated with FLAG beads. Co-IP and IP were immunoblotted with antibodies against GRK2 and FLAG epitope. Lysates were immunoblotted with antibodies against lamin and β-actin. DA(+)/CF group (1.0 ± 0.0) was significantly different from DA(-)/CF group (2.4 ± 0.65) (p < 0.01, n = 3). (B) HEK-293 cells expressing D2R (1.9–2.1 pmol/mg protein) were transfected with arrestin3-GFP. Cells were treated with 20 nM LMB for 3 hr. The γ value between DAPI and arrestin3 changed from 0.27 ± 0.17 to 0.58 ± 0.25 (p< 0.01, n = 6) upon LMB treatment. The horizontal bars represent 10 μm. (C) HEK-293 cells expressing D2R (1.9–2.1 pmol/mg protein) were transfected with GRK2-GFP. Cells were treated with 20 nM LMB for 7 hr. This image represents a typical example of six cells exhibiting comparable patterns. The γ value did not change significantly with LMB treatment. The horizontal bars represent 10 μm.
Figure 1.
Roles of Mdm2-mediated GRK2 ubiquitination that occurs in the cytoplasm. (A) HEK-293 cells expressing β2AR or D2R (1.9–2.1 pmol/mg protein) were transfected with GRK2 and FLAG-Mdm2. Subcellular fractionation was conducted into the nuclear fraction (NF) and cytoplasmic fraction (CF), and each fraction was immunoprecipitated with FLAG beads. Co-IP and IP were immunoblotted with antibodies against GRK2 and FLAG epitope. Lysates were immunoblotted with antibodies against lamin and β-actin. DA(+)/CF group (1.0 ± 0.0) was significantly different from DA(-)/CF group (2.4 ± 0.65) (p < 0.01, n = 3). (B) HEK-293 cells expressing D2R (1.9–2.1 pmol/mg protein) were transfected with arrestin3-GFP. Cells were treated with 20 nM LMB for 3 hr. The γ value between DAPI and arrestin3 changed from 0.27 ± 0.17 to 0.58 ± 0.25 (p< 0.01, n = 6) upon LMB treatment. The horizontal bars represent 10 μm. (C) HEK-293 cells expressing D2R (1.9–2.1 pmol/mg protein) were transfected with GRK2-GFP. Cells were treated with 20 nM LMB for 7 hr. This image represents a typical example of six cells exhibiting comparable patterns. The γ value did not change significantly with LMB treatment. The horizontal bars represent 10 μm.
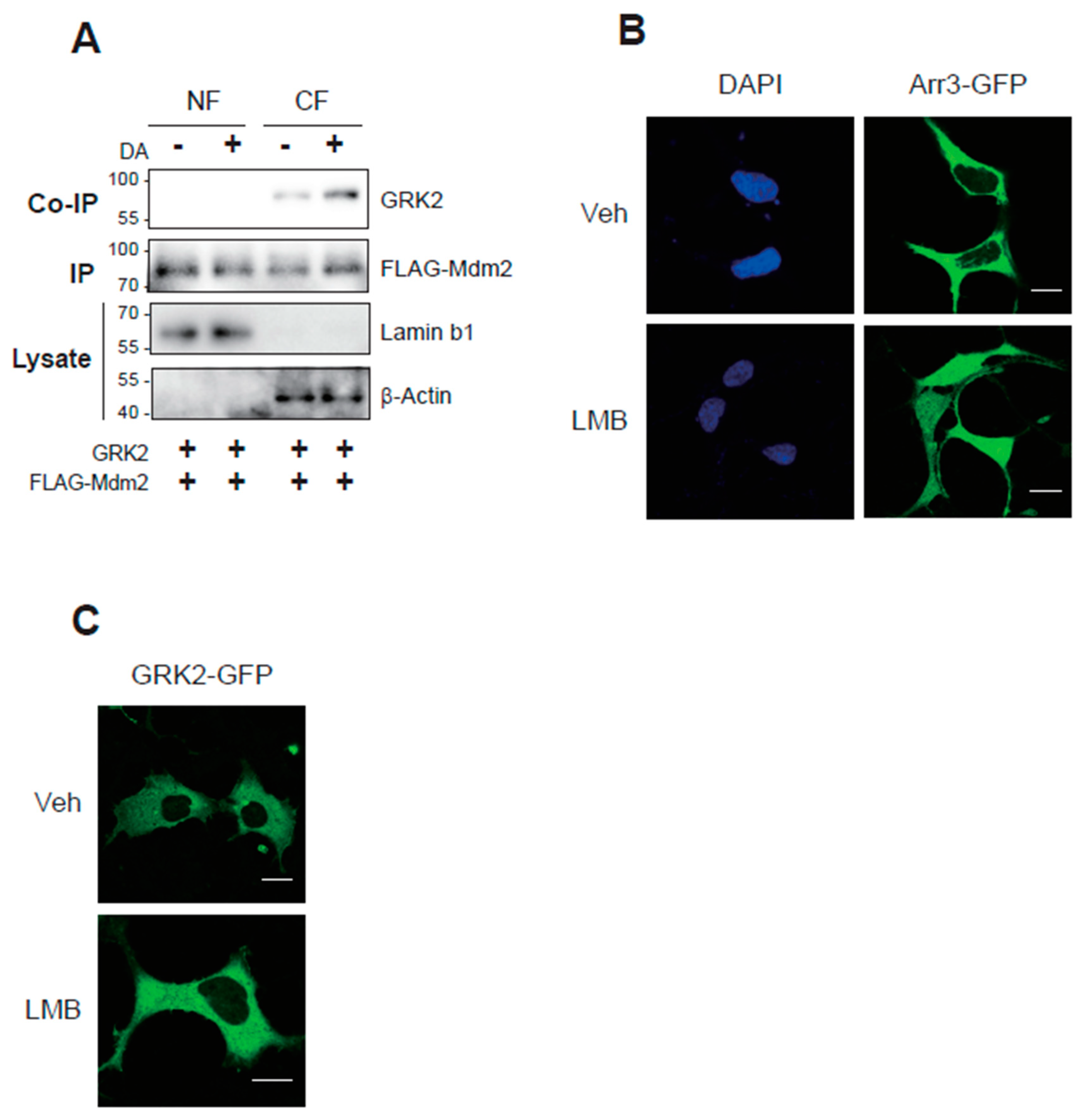
Figure 2.
The ubiquitination of arrestin3 is needed for the ubiquitination of GRK2. (A) Con-KD and arrestin2/3-KD HEK-293 cells were transfected with D2R (1.7–2.1 pmol/mg protein), HA-Ub, and FLAG-tagged GRK2. Cells were treated with 10 μM DA for 2 min and ubiquitination assay was conducted. The knockdown efficiency of arrestin2 and arrestin3 was about 90%. **p < 0.01 compared to other groups (n = 3). (B) Con-KD and arrestin2/3-KD cells were transfected with GRK2 and FLAG-Mdm2 along with WT- or K11/12R-arrestin3. Cells were treated with 10 μM DA for 2 min. Cell lysates were immunoprecipitated with agarose beads coated with antibodies against the FLAG epitope. Co-IP/lysates and IPs were immunoblotted with antibodies against GRK2 and FLAG epitope. *P < compared to corresponding Veh group (n = 3).
Figure 2.
The ubiquitination of arrestin3 is needed for the ubiquitination of GRK2. (A) Con-KD and arrestin2/3-KD HEK-293 cells were transfected with D2R (1.7–2.1 pmol/mg protein), HA-Ub, and FLAG-tagged GRK2. Cells were treated with 10 μM DA for 2 min and ubiquitination assay was conducted. The knockdown efficiency of arrestin2 and arrestin3 was about 90%. **p < 0.01 compared to other groups (n = 3). (B) Con-KD and arrestin2/3-KD cells were transfected with GRK2 and FLAG-Mdm2 along with WT- or K11/12R-arrestin3. Cells were treated with 10 μM DA for 2 min. Cell lysates were immunoprecipitated with agarose beads coated with antibodies against the FLAG epitope. Co-IP/lysates and IPs were immunoblotted with antibodies against GRK2 and FLAG epitope. *P < compared to corresponding Veh group (n = 3).
As shown in
Figure 3A,B, sequestration of Gβγ or knockdown of clathrin inhibited the ubiquitination of GRK2. Finally, a mutant of β
2AR, GRK2-KO-β
2AR, in which GRK2-mediated phosphorylation sites (T360, S364, S396, S401, S407, S411) were altered, failed to mediate GRK2 ubiquitination (
Figure 3C), suggesting that receptor phosphorylation is also involved in the ubiquitination of GRK2.
Based on these results, we next investigated how these cellular components regulate the nuclear export of Mdm2, which is a critical process necessary for the ubiquitination of GRK2.
2.3. Arrestin3 Shuttles between the Nucleus and Cytosol, Controlling the Subcellular Localization of Mdm2 and the Ubiquitination of GRK2
Because the translocation of Mdm2 from the nucleus to the cytosol is necessary for the ubiquitination of cytosolic proteins, we examined whether the cellular components shown in
Figure 3 are involved in the nuclear export of Mdm2.
Initially, we investigated the impact of arrestins on the subcellular trafficking of Mdm2. As depicted in
Figure 4A, under basal conditions, Mdm2 was primarily localized in the nucleus in cells expressing D
2R, regardless of arrestin knockdown (Veh-treated groups). However, upon dopamine (DA) treatment, Mdm2 translocated from the nucleus to the cytosolic region (
Figure 4A, DA-treated/Con-KD). Notably, this translocation was impeded by cellular arrestin knockdown (DA-treated/Arr-KD).
Because the agonist-induced cytosolic translocation of Mdm2 occurs in an arrestin-dependent manner, we were curious whether the trafficking properties of arrestin3 are associated with its ability to facilitate the agonist-induced nuclear export of Mdm2. For this, we utilized the mutants of arrestin3 targeting its nuclear localization signal (NLSX) [
18] and nuclear export signal (NESX, L395A) [
19]. In NLSX-arrestin3, seven amino acid residues (158–162 and 170–171) were mutated to alanine.
As illustrated in
Figure 4B,C, co-expression of WT-arrestin3, but not NLSX- or NESX-arrestin3, restored GRK2 ubiquitination. These findings, along with the results shown in
Figure 2B, indicate that the nuclear entry of arrestin3, its interaction with Mdm2 in the nucleus, and the subsequent nuclear export of arrestin3 are crucial for the D
2R activation-mediated nuclear export of Mdm2, which is necessary for GRK2 ubiquitination.
This assumption was further supported by two experiments. First, GRK2 ubiquitination was inhibited when cells were treated with LMB (
Figure 5A), which inhibits CRM1 and blocks the nuclear export of proteins [
20]. Next, we examined the nucleocytoplasmic shuttling of arrestin3 and Mdm2 (
Figure 5B). Approximately 1 min after DA treatment, arrestin3 moved from the cytosol to the nucleus and then returned to the cytoplasm about 5 min after DA treatment. Mdm2 started moving from the nucleus to the cytoplasm about 3 min after DA treatment.
2.4. Gβγ and Clathrin Regulate the Nuclear Shuttling of Arrestin3 that Determines the Nuclear Export of Mdm2
Gβγ and clathrin play roles in the ubiquitination of GRK2 (
Figure 3A,B), while arrestins are particularly crucial, as they shuttle between the cytoplasm and nucleus [
8]. Clathrin constitutes a major component of coat proteins found on clathrin-mediated endocytic vesicles [
21,
22]. To elucidate their roles in the nuclear shuttling of Mdm2 and arrestin3, we examined the influence of Gβγ and the knockdown of clathrin heavy chain (CHC) on the nucleocytoplasmic shuttling of Mdm2 and arrestin3.
Under basal conditions, Mdm2 was predominantly localized in the nucleus (
Figure 6A, Veh/Mock). Agonistic activation of D
2R caused Mdm2 to relocate from the nucleus to the cytoplasm (DA/Mock). However, co-expression of GRK2-CT, which sequesters Gβγ, prevented this agonist-induced cytoplasmic translocation of Mdm2 (GRK2-CT). As depicted in
Figure 6B in the Mock/CHC-KD panel, knocking down CHC did not alter the subcellular distribution of Mdm2 and D
2R, but it did prevent the DA-induced nuclear export of Mdm2.
In its basal state, arrestin3 was primarily located in the cytosol (
Figure 6C, Veh/Mock). However, upon DA stimulation following LMB pretreatment (LMB∙DA/Mock), a portion of arrestin3 translocated to the nucleus. This translocation was partially inhibited by sequestering Gβγ (LMB∙DA/GRK2-CT) and more extensively hindered by CHC knockdown (LMB∙DA/CHC-KD).
These findings suggest that Gβγ and clathrin are essential for the nuclear entry of arrestin3, which consequently influences the nuclear export of Mdm2.
2.5. Receptor Phosphorylation Mediate arrestin3 Ubiquitination and Nuclear Export of Mdm2 That Are Needed for the GRK2 Ubiquitination
Next, we determined the roles of receptor phosphorylation in the ubiquitination of GRK2. For this, GRK2-KO-β
2AR in which the consensus GRK2 phosphorylation sites are mutated [
23], and D
2R-IC23 in which all of the serine and threonine residues in the 2nd and 3rd intracellular loops are mutated [
24] were utilized.
As shown in
Figure 7A, GRK2-KO-β
2AR, which failed to mediate GRK2 ubiquitination (
Figure 3C), also could not mediate arrestin3 ubiquitination. Agonistic stimulation of D
2R caused the nuclear export of Mdm2 (
Figure 7B, compare Veh- and DA-treated cells expressing WT-D
2R). In contrast, D
2R-IC23 failed to mediate the nuclear export of Mdm2 (cells expressing D
2R-IC23).
These results suggest that receptor phosphorylation is necessary for the ubiquitination of arrestin3 and the nuclear export of Mdm2, both of which are required for GRK2 ubiquitination. Furthermore, the ubiquitination of GRK2 is necessary for its interaction with the receptor (
Figure 1D), which is required for receptor phosphorylation.
Figure 6.
Roles of Gβγ and CHC in the nucleocytoplasmic translocation of Mdm2 and arrestin3. The results represent similar results from seven different cells. The horizontal bars represent 10 μm. (A) HEK-293 cells were transfected with Mdm2-GFP along with a mock vector or GRK2-CT. Cells were treated with either vehicle or 10 μM DA for 2 min. In the mock-transfected cells, the ratio of the space where Mdm2 is observed and the space surrounded by FLAG-D2R increased from 0.24 ± 0.08 to 0.92 ± 0.15 (p < 0.001, n = 7). In the cells transfected with GRK2-CT, the ratios were 0.34 ± 0.17 and 0.37 ± 0.12 (n = 7) for Veh and DA groups, respectively. (B) Con-KD and CHC-KD HEK-293 cells were transfected with Mdm2-GFP. Cells were treated with either vehicle or 10 μM DA for 2 min. In the mock-transfected cells, the ratio of the space where Mdm2 is observed and the space surrounded by FLAG-D2R increased from 0.21 ± 0.07 to 0.89 ± 0.13 (p < 0.001, n = 7). In the CHC-KD cells, the ratios were 0.24 ± 0.18 and 0.27 ± 0.17 (n = 7) for the Veh and DA groups, respectively. (C) Con-KD HEK-293 cells were transfected with arrestin3-GFP along with a mock vector or GRK2-CT (upper 8 panels); Con-KD and CHC-KD HEK-293 cells were transfected with arrestin3-GFP (left 8 panels). Cells were treated with either vehicle or 20 nM LMB for 3hr, followed by 10 μM DA for 2 min. In the Mock/Con-KD cells, the ratio of the space where Mdm2 is observed and the space surrounded by FLAG-D2R increased from 0.19 ± 0.08 to 0.87 ± 0.15 (p < 0.001, n = 8). In the GRK2-CT/Con-KD cells, the ratio increased from 0.21 ± 0.12 and 0.56 ± 0.42 (p < 0.05, n = 8) for Veh and LMB/DA groups, respectively. In the Mock/CHC-KD cells, the ratios were 0.19 ± 0.08 and 0.27 ± 0.18 (p < 0.05, n= 10) for Veh and DA/LMB groups, respectively.
Figure 6.
Roles of Gβγ and CHC in the nucleocytoplasmic translocation of Mdm2 and arrestin3. The results represent similar results from seven different cells. The horizontal bars represent 10 μm. (A) HEK-293 cells were transfected with Mdm2-GFP along with a mock vector or GRK2-CT. Cells were treated with either vehicle or 10 μM DA for 2 min. In the mock-transfected cells, the ratio of the space where Mdm2 is observed and the space surrounded by FLAG-D2R increased from 0.24 ± 0.08 to 0.92 ± 0.15 (p < 0.001, n = 7). In the cells transfected with GRK2-CT, the ratios were 0.34 ± 0.17 and 0.37 ± 0.12 (n = 7) for Veh and DA groups, respectively. (B) Con-KD and CHC-KD HEK-293 cells were transfected with Mdm2-GFP. Cells were treated with either vehicle or 10 μM DA for 2 min. In the mock-transfected cells, the ratio of the space where Mdm2 is observed and the space surrounded by FLAG-D2R increased from 0.21 ± 0.07 to 0.89 ± 0.13 (p < 0.001, n = 7). In the CHC-KD cells, the ratios were 0.24 ± 0.18 and 0.27 ± 0.17 (n = 7) for the Veh and DA groups, respectively. (C) Con-KD HEK-293 cells were transfected with arrestin3-GFP along with a mock vector or GRK2-CT (upper 8 panels); Con-KD and CHC-KD HEK-293 cells were transfected with arrestin3-GFP (left 8 panels). Cells were treated with either vehicle or 20 nM LMB for 3hr, followed by 10 μM DA for 2 min. In the Mock/Con-KD cells, the ratio of the space where Mdm2 is observed and the space surrounded by FLAG-D2R increased from 0.19 ± 0.08 to 0.87 ± 0.15 (p < 0.001, n = 8). In the GRK2-CT/Con-KD cells, the ratio increased from 0.21 ± 0.12 and 0.56 ± 0.42 (p < 0.05, n = 8) for Veh and LMB/DA groups, respectively. In the Mock/CHC-KD cells, the ratios were 0.19 ± 0.08 and 0.27 ± 0.18 (p < 0.05, n= 10) for Veh and DA/LMB groups, respectively.
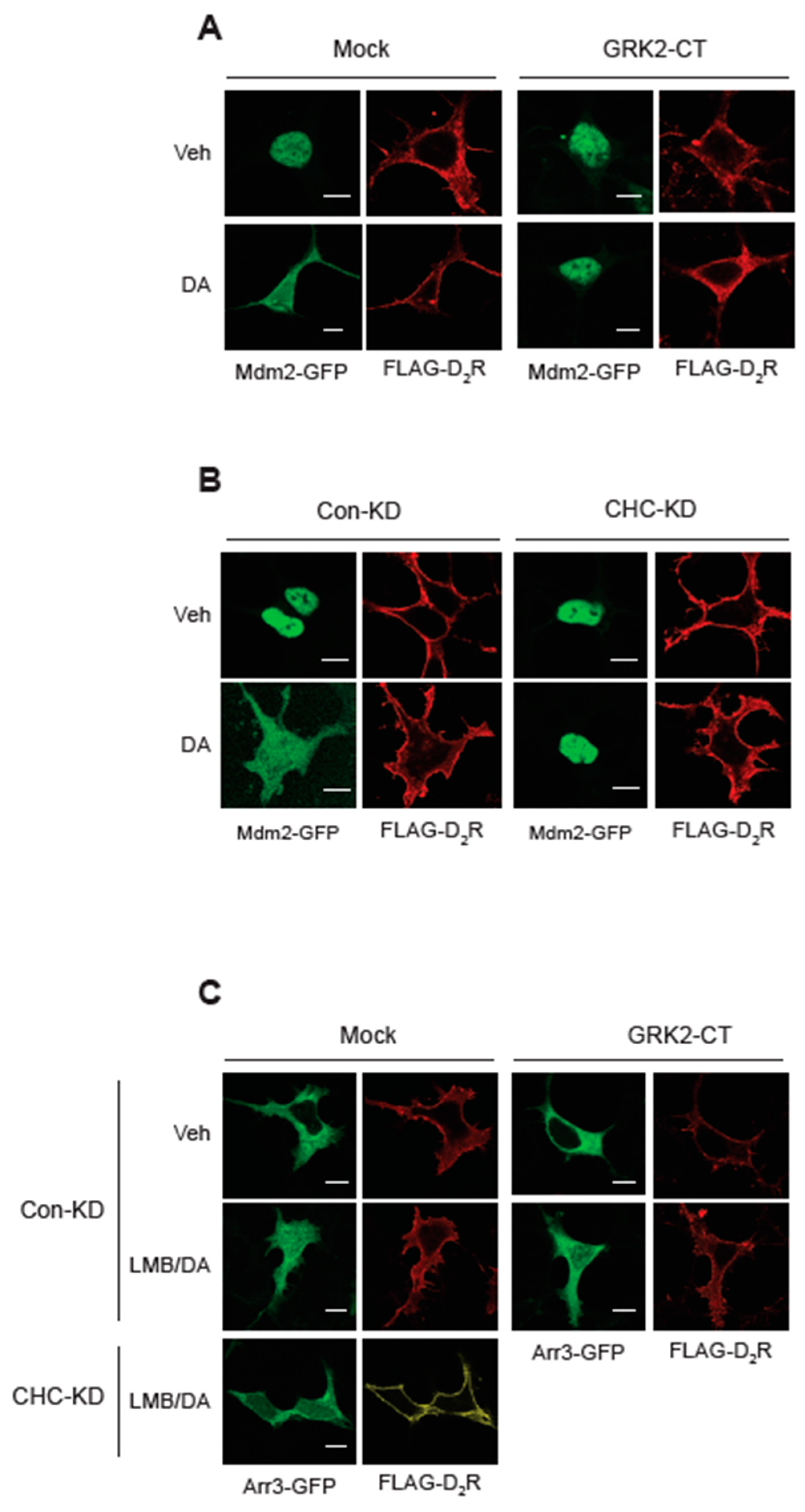
Figure 7.
Roles of receptor phosphorylation on the ubiquitination of arrestin3 and nuclear export of Mdm2. (A) HEK-293 cells were transfected with HA-Ub and FLAG-arrestin3 along with WT-β2AR or GRK2-KO-β2AR. Cells were treated with 10 μM isoproterenol for 2 min. Ubiquitination was conducted as described above. *p < 0.05 compared to other groups (n = 3). (B) HEK-293 cells were transfected with Mdm2-GFP along with WT-D2R or D2R-IC23. Cells were treated with either vehicle or 10 μM DA for 2 min. In the WT-D2R expressing cells, the γ value between DAPI and Mdm2 changed from 0.95 ± 0.12 to 0.52 ± 0.15 (p< 0.001, n = 7) upon DA treatment. In the cells expressing IC23-D2R expressing cells, the γ values were 0.93 ± 0.17 to 0.92 ± 0.15 for the vehicle- and DA-treated cells (n = 7). The horizontal bars represent 10 μm.
Figure 7.
Roles of receptor phosphorylation on the ubiquitination of arrestin3 and nuclear export of Mdm2. (A) HEK-293 cells were transfected with HA-Ub and FLAG-arrestin3 along with WT-β2AR or GRK2-KO-β2AR. Cells were treated with 10 μM isoproterenol for 2 min. Ubiquitination was conducted as described above. *p < 0.05 compared to other groups (n = 3). (B) HEK-293 cells were transfected with Mdm2-GFP along with WT-D2R or D2R-IC23. Cells were treated with either vehicle or 10 μM DA for 2 min. In the WT-D2R expressing cells, the γ value between DAPI and Mdm2 changed from 0.95 ± 0.12 to 0.52 ± 0.15 (p< 0.001, n = 7) upon DA treatment. In the cells expressing IC23-D2R expressing cells, the γ values were 0.93 ± 0.17 to 0.92 ± 0.15 for the vehicle- and DA-treated cells (n = 7). The horizontal bars represent 10 μm.
2.6. Importin Complex is Involved in the Nuclear Import of Mdm2 and Arrestin3
The nuclear movement of cytosolic protein molecules is commonly explained by the classical nuclear import pathway that employs importin α/β complex [
25,
26]. In this pathway, a protein containing a classical basic NLS, which acts as a cargo, binds to the importin α, an adaptor protein. In collaboration with importin β, which binds to the cargo and mediates interactions with the nuclear pore complex, the cargo is transferred to Ran GTP in the nucleus [
27,
28]. Mdm2 possesses a clear classical NLS sequence [
14]. Arrestin3 also possesses an NLS sequence, and it was suggested that arrestin3 enters the nucleus through binding with another protein that possesses an NLS sequence [
29]. Thus, it is likely that Mdm2 and arrestin3 have similar but somewhat different regulatory mechanisms for their nuclear entry.
Arrestin3, which was located in the cytosol (
Figure 8A, left/upper panel), was observed both in the cytosol and nucleus when cells were treated with LMB (
Figure 8A, left/lower panel). When cellular importin β1 was knocked down, arrestin3 largely failed to translocate to the nucleus (
Figure 8A, right panels).
Mdm2, which was located within the nucleus at the basal state (
Figure 8B, Veh/Con-KD), was located both in the nucleus and cytosol after DA treatment (DA/Con-KD). Upon knockdown of cellular importin β1, the localization of Mdm2 was detected in both the cytosol and nucleus at basal state (
Figure 8B, Veh/Impo-KD cells), suggesting that a portion of Mdm2’s nuclear entry under basal conditions was compromised. Because Mdm2 was already situated in the cytoplasm under basal conditions in importin β1-KD cells, it was challenging to conclusively ascertain whether nuclear export was further augmented by DA treatment.
Subsequently, we investigated whether the nucleo-cytoplasmic shuttling of arrestin3 and Mdm2 mediated by the importin1 complex is implicated in GRK2 ubiquitination in the cytoplasm. As depicted in
Figure 8C, activation of D
2R resulted in GRK2 ubiquitination, which was hindered by importin β1 knockdown.
These findings highlight that the nuclear entry of arrestin3 and Mdm2 facilitated by the importin1 complex, along with the ubiquitination of arrestin3 within the nucleus via this mechanism, are pivotal processes for GRK2 ubiquitination in the cytoplasm.
2.7. Interaction between Importin β1 and Arrestin3 is Supported by Gβγ, Clathrin, and Receptor Phosphorylation
According to our findings, the ubiquitination of GRK2 relies on the cytosolic translocation of Mdm2, which, in turn, is contingent upon the interaction between arrestin3 and Mdm2 within the nucleus. This process is facilitated by the nuclear entry of arrestin3 through the importin complex. As Gβγ, clathrin, and receptor phosphorylation are pivotal for GRK2 ubiquitination, we investigated their potential involvement in the final phase of the cascade responsible for GRK2 ubiquitination—specifically, the nuclear entry of arrestin3 facilitated by the importin complex.
As anticipated, the interaction between importin β1 and arrestin3 increased upon agonist stimulation of D
2R. However, this augmented interaction was nullified by sequestering Gβγ (
Figure 9A). Additionally, the knockdown of clathrin, but not caveolin1, also hindered the interaction (
Figure 9B). Finally, the impact of receptor phosphorylation on their interaction was assessed using D
2R-IC23. The augmented interaction between importin β1 and arrestin3 triggered by agonist stimulation of D
2R was not observed with D
2R-IC23, a mutant lacking phosphorylation sites [
24] (
Figure 9C).
In summary, these findings highlight that the ubiquitination of GRK2 by Mdm2 is regulated by the translocation of arrestin3 between the nucleus and cytosol, along with its interaction with Mdm2 within the nucleus. Gβγ, clathrin, and receptor phosphorylation play crucial roles in facilitating these processes.
Figure 8.
Involvement of importin complex in the nuclear entry of arrestin3 and Mdm2. (A) The results represent similar results from seven different cells. The horizontal bars represent 10 μm. (B) Con-KD and importin β1-KD HEK-293 cells were transfected with arrestin3-GFP. Cells were treated with either vehicle or 20 nM LMB for 8 hr. In the Con-KD cells, the γ value between DAPI and arrestin3 changed from 0.21 ± 0.08 to 0.52 ± 0.12 (p < 0.001, n = 7) upon LMB treatment. In the importin β1-KD cells, the γ values between DAPI and arrestin3 were 0.17 ± 0.07 to 0.24 ± 0.13 (n = 7) for the vehicle- and LMB-treated cells, respectively. The horizontal bars represent 10 μm. The knockdown efficiency of importin β1 was about 93%. (C) Con-KD and importin β1-KD HEK-293 cells expressing D2R were transfected with Mdm2-GFP. Cells were treated with either vehicle or 10 μM DA. In the Con-KD cells, the γ value between DAPI and Mdm2 changed from 0.92 ± 0.18 to 0.57 ± 0.22 (p < 0.001, n = 7) upon LMB treatment. In the importin β1-KD cells, the γ values between DAPI and Mdm2 were 0.87 ± 0.17 to 0.91 ± 0.23 (n = 7) for the vehicle- and LMB-treated cells, respectively. The horizontal bars represent 10 μm. (D) Con-KD and importin β1-KD cells expressing D2R were transfected with HA-Ub and FLAG-GRK2. Cells were treated with 10 μM DA. **p < 0.01 compared to other groups (n = 3).
Figure 8.
Involvement of importin complex in the nuclear entry of arrestin3 and Mdm2. (A) The results represent similar results from seven different cells. The horizontal bars represent 10 μm. (B) Con-KD and importin β1-KD HEK-293 cells were transfected with arrestin3-GFP. Cells were treated with either vehicle or 20 nM LMB for 8 hr. In the Con-KD cells, the γ value between DAPI and arrestin3 changed from 0.21 ± 0.08 to 0.52 ± 0.12 (p < 0.001, n = 7) upon LMB treatment. In the importin β1-KD cells, the γ values between DAPI and arrestin3 were 0.17 ± 0.07 to 0.24 ± 0.13 (n = 7) for the vehicle- and LMB-treated cells, respectively. The horizontal bars represent 10 μm. The knockdown efficiency of importin β1 was about 93%. (C) Con-KD and importin β1-KD HEK-293 cells expressing D2R were transfected with Mdm2-GFP. Cells were treated with either vehicle or 10 μM DA. In the Con-KD cells, the γ value between DAPI and Mdm2 changed from 0.92 ± 0.18 to 0.57 ± 0.22 (p < 0.001, n = 7) upon LMB treatment. In the importin β1-KD cells, the γ values between DAPI and Mdm2 were 0.87 ± 0.17 to 0.91 ± 0.23 (n = 7) for the vehicle- and LMB-treated cells, respectively. The horizontal bars represent 10 μm. (D) Con-KD and importin β1-KD cells expressing D2R were transfected with HA-Ub and FLAG-GRK2. Cells were treated with 10 μM DA. **p < 0.01 compared to other groups (n = 3).
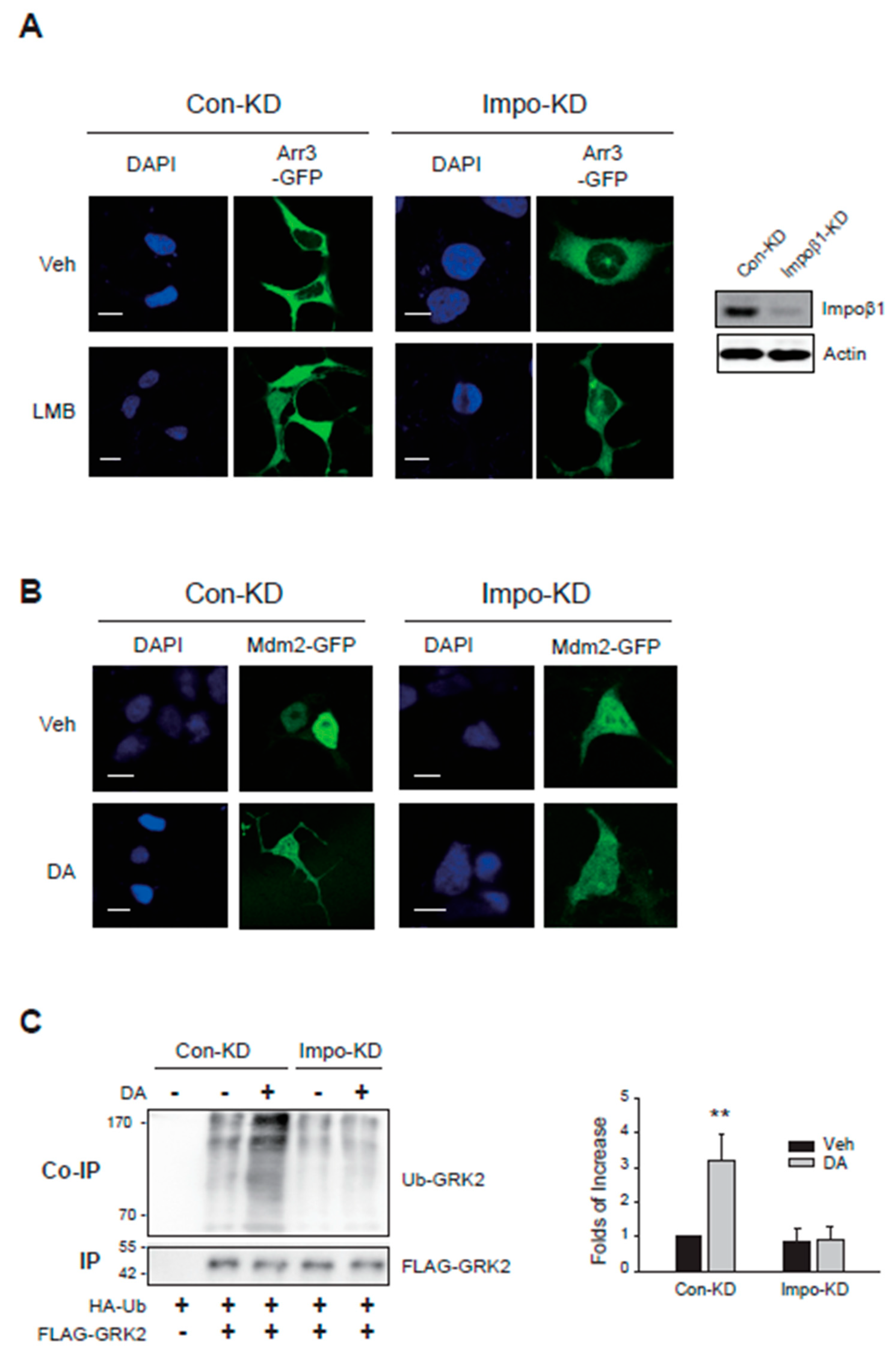
Figure 9.
Roles of Gβγ, CHC, and receptor phosphorylation on the interaction between importin β1 and arrestin3. (A) HEK-293 cells expressing D2R were transfected with FLAG-arrestin3 along with a mock vector or GRK2-CT. Cells were treated with 10 μM DA for 2 min. Cell lysates were immunoprecipitated with FLAG beads. Co-IP/lysates and IPs were immunoblotted with antibodies against importin β1 and FLAG, respectively. *p < 0.05 compared to the Veh/Mock group (n = 3). (B) Con-KD, CHC-KD, and Cav1-KD HEK-293 cells transfected with D2R and FLAG-arrestin3. Cells were treated with either vehicle or 10 μM DA for 2 min. *p < 0.05 compared to the corresponding vehicle-treated group and CHC-KD cells (n = 3). (C) HEK-293 cells were transfected with FLAG-arrestin3 together with WT-D2R or D2R-IC23. Cells were treated with 10 μM DA for 2 min. *p < 0.05 compared to other groups (n = 3).
Figure 9.
Roles of Gβγ, CHC, and receptor phosphorylation on the interaction between importin β1 and arrestin3. (A) HEK-293 cells expressing D2R were transfected with FLAG-arrestin3 along with a mock vector or GRK2-CT. Cells were treated with 10 μM DA for 2 min. Cell lysates were immunoprecipitated with FLAG beads. Co-IP/lysates and IPs were immunoblotted with antibodies against importin β1 and FLAG, respectively. *p < 0.05 compared to the Veh/Mock group (n = 3). (B) Con-KD, CHC-KD, and Cav1-KD HEK-293 cells transfected with D2R and FLAG-arrestin3. Cells were treated with either vehicle or 10 μM DA for 2 min. *p < 0.05 compared to the corresponding vehicle-treated group and CHC-KD cells (n = 3). (C) HEK-293 cells were transfected with FLAG-arrestin3 together with WT-D2R or D2R-IC23. Cells were treated with 10 μM DA for 2 min. *p < 0.05 compared to other groups (n = 3).
3. Discussion
GRK2 and arrestins are central to the regulation and signaling of GPCRs [
1,
3]. Both GRK2 and arrestin3 are targets of ubiquitination by Mdm2, where arrestin3 ubiquitination plays a crucial role in regulatory processes such as arrestin3 downregulation and receptor endocytosis [
6]. Similarly, GRK2 undergoes ubiquitination by Mdm2, contributing to GRK2 downregulation [
7]. In the case of arrestin3, it has been observed that upon agonistic activation of GPCRs, there is an augmentation in the translocation of cytosolic arrestin3 into the nucleus, thereby facilitating Mdm2-mediated ubiquitination [
8]. Given that GRK2 lacks an NLS and mainly resides in the cytoplasm, the relocation of Mdm2 from the nucleus to the cytoplasm becomes essential for GRK2 ubiquitination. Our research was conducted with a focus on the regulatory pathways associated with the ubiquitination of arrestin3, which is closely intertwined with the nucleocytoplasmic translocation of Mdm2.
A typical model explaining GRK2 activation involves the following steps: Initially, an agonist binds to the GPCR, leading to the activation of the receptor and the dissociation of the associated trimeric GTP-binding proteins into Gα and Gβγ subunits. The free Gβγ subunit, along with plasma membrane phospholipids, then recruits inactive GRK2 to the activated GPCR, allosterically activating GRK2 and enabling it to phosphorylate the receptors.
The overall process of GRK2 ubiquitination triggered by agonist stimulation of GPCRs is depicted in
Figure 10. Fundamentally, the critical step determining cytosolic GRK2 ubiquitination appears to be step 5, involving the movement of Mdm2 from the nucleus to the cytoplasm.
According to our experimental results, arrestin (
Figure 2A), especially its ubiquitination (
Figure 2B), is required for the ubiquitination of GRK2. Hence, it could be inferred that the factors governing the nuclear entry of arrestin3 (steps 1 and 2), crucial for its ubiquitination, would likewise influence the ubiquitination of GRK2. This hypothesis was indeed confirmed by our study findings (
Figure 5 and
Figure 8). As Mdm2 is a nuclear protein, and both arrestin3 and Mdm2 contain NES, it is presumed that Mdm2 and ubiquitinated arrestin3 relocate from the nucleus to the cytoplasm driven by the concentration gradient (step 4,
Figure 5B).
Reportedly, clathrin is required for GRK2-mediated receptor phosphorylation [
30]. This function of clathrin was difficult to anticipate due to its typical role in facilitating receptor endocytosis [
31,
32]. However, it can now be elucidated by clathrin’s participation in the ubiquitination of GRK2 (
Figure 3B). This is one piece of evidence supporting the idea that clathrin has functions beyond simply being a coated protein of endocytic vesicles. This evidence suggests that clathrin possesses functions extending beyond its conventional role as a coated protein of endocytic vesicles.
According to previous reports, the cytosolic localization of Mdm2 can result from the overexpression of arrestin3 [
29] or the presence of GPCRs with a high affinity for Gβγ [
33,
34]. This phenomenon is significant as it may relate to GPCR desensitization characteristics [
35,
36], but it is observed in the basal state without GPCR activation. In contrast, the increase in nuclear ubiquitination of arrestin3 discussed in this study occurs in a dynamic situation induced by agonistic stimulation of GPCRs.
Arrestin-3 has previously been shown to be ubiquitinated when bound to a GPCR on the plasma membrane [
6]. While this appears to conflict with our results, the discrepancy lies in the timing of arrestin3 ubiquitination. The earlier study did not consider the interaction between arrestin3 and Mdm2 in the nucleus, leading them to observe ubiquitination at the cytosolic interface of the plasma membrane.
4. Materials and Methods
4.1. Materials
Leptomycin B (LMB), dopamine (DA), isoproterenol (ISO), MβCD, 4′,6-diamidine-2′-phenylindole (DAPI), methyl-β-Cyclodextrin (MβCD), rabbit FLAG antibodies, and antibodies against β-actin, agarose beads coated with anti-M2 FLAG antibodies, and anti-mouse or anti-rabbit horse radish peroxidase (HRP)-labeled secondary antibodies were obtained from Sigma-Aldrich Chemical Co. (St. Louis, MO, USA). Mouse monoclonal antibodies against clathrin heavy chain (CHC) and caveolin 1 (Cav1), and rabbit antibodies against lamin b1 were purchased from BD Biosciences (San Jose, CA, USA) and BD Transduction Laboratories (Franklin Lakes, NJ, USA), respectively. Monoclonal antibodies against hemagglutinin (HA), Mdm2, and importin β1 were purchased from Santa Cruz Biotechnology (Dallas, TX, USA). Rabbit antibodies against arrestin 2/3 were purchased from Cell Signaling Technology (Danvers, MA, USA). Alexa 594-conjugated anti-rabbit antibodies and Alexa 647-conjugated anti-rabbit antibodies were purchased from Thermo Fisher Scientific (Waltham, MA, USA). Anti-rabbit HRP-conjugated secondary antibodies were purchased from Thermo Fisher Scientific (Waltham, MA, USA). Anti-mouse HRP-conjugated secondary antibodies were obtained from Jackson ImmunoResearch (West Grove, PA, USA). [3H]-Methylspiperone and [3H]-CGP-12177 (41.7 Ci/mmol) were purchased from PerkinElmer Life Sciences (Waltham, MA, USA).
4.2. DNA Constructs
HA-Ub, FLAG-arrestin3, arrestin3-GFP, K11/12R-arrestin3-GFP, NLSX-arrestin3-GFP, NESX (L395A)-arrestin3-GFP, GRK2, FLAG-GRK2, GRK2-GFP, FLAG-Mdm2, Mdm2-GFP, GRK2-CT, D
2R, FLAG-D
2R, D
2R-IC23, β
2AR, and GRK2-KO-β
2AR were described previously [
8,
22,
23,
24,
37]. In, GRK2-KO-β
2AR, the consensus phosphorylation sites for GRK2 (T360, S364, S396, S401, S407, S411) were mutated [
23]. In D
2R-IC23, all the serine and threonine residues located within the second and third intracellular loops of D
2R were mutated to alanine and valine residues, respectively [
24]. This mutant has been used to study phosphorylation-independent receptor functions previously [
38].
4.3. Cell Culture
Human embryonic kidney (HEK-293) cells were obtained from the American Type Culture Collection (Manassas, VA, USA) and maintained in minimal essential medium supplemented with 10% fetal bovine serum, 100 units/mL penicillin, and 100 μg/mL streptomycin (Thermo Fisher Scientific) in a humidified atmosphere at 5% CO
2. Mdm2-knockdown (KD) cells, CHC-KD cells, Cav1-KD cells, and importin β1-KD cells were prepared by a selection of the cells stably transfected with shRNA in pLKO.1 using 1 μg/mL puromycin [
9,
22,
39,
40]. Arrestin2/3-KD cells were prepared by simultaneous transfection of shRNAs targeted against arrestin2 and arrestin3; the transfected cells were selected using 500 μg/mL G418 and 1 μg/mL zeocin [
40].
4.4. Immunoprecipitation and Immunoblotting
For immunoprecipitation, target proteins tagged with FLAG epitope were expressed in HEK-293 cells. The cells were lysed using either RIPA buffer (150 mM NaCl, 50 mM Tris pH 8.0, 1% NP-40, 0.5% deoxycholate, and 0.1% SDS) or glycerol lysis buffer (10 mM HEPES, pH 7.4, 150 mM NaCl, 10% glycerol, and 0.1% NP-40) for 1 h at 4°C. Subsequently, the cell lysates were centrifuged for 30 min at 14,000 ×g. The resulting supernatants were combined with 25 μL of anti-FLAG agarose beads (50% slurry) and incubated for 2–3 hours at 4°C. The beads underwent three washes with ice-cold washing buffer (50 mM Tris pH 7.4, 137 mM NaCl, 10% glycerol, and 1% NP-40), each lasting 5 minutes.
Immunoprecipitates were then subjected to analysis on SDS-PAGE gels and transferred onto nitrocellulose membranes (Thermo Fisher Scientific). The membranes were subjected to overnight incubation at 4°C with primary antibodies against the target proteins, followed by a 1 h incubation at 20°C with the corresponding HRP-conjugated secondary antibodies. Visualization of protein bands was achieved using a chemiluminescent HRP substrate (Thermo Fisher Scientific). The detection of target proteins was accomplished with a chemiluminescent substrate, and the immunoblots were quantified using the ChemiDoc MP imaging system from (BioRad, Hercules, CA, USA).
4.5. Immunocytochemistry
Cells expressing the relevant target proteins were seeded onto coverslips and fixed with a solution comprising 4% paraformaldehyde and 0.2% Triton X-100 in PBS, maintaining this fixation for 20 min at 20°C. Subsequently, the cells underwent incubation with PBS containing 3% FBS and 1% bovine serum albumin for 1 h, followed by a 1 h incubation with antibodies specific to the target proteins at 20°C. Following three washes, the cells were subjected to incubation with Alexa 595-conjugated anti-rabbit or anti-mouse antibody (Invitrogen, Carlsbad, CA, USA) diluted at 1:500. After another three washes, the cells were mounted on slides using Vectashield (Vector Laboratories, Burlingame, CA, USA) and visualized using a laser scanning confocal microscope (TCS SP5/AOBS/Tandem, Leica Microsystems GmbH, Wetzlar, Germany). A range of 5 to 10 cells were analyzed for each experimental group.
4.6. Image Processing
The images were imported into the Fiji version of the ImageJ image processing software. The assessment of spatial overlap among distinct fluorescent labels (co-localization) was conducted utilizing the Pearson correlation coefficient (γ value) [
41]. Subsequently, the level of co-localization was categorized into three groups: strong (γ value ranging from 0.50 to 1), moderate (0.30 to 0.49), and weak (below 0.29).
4.7. Detection of Protein Ubiquitination
HA-ubiquitin (HA-Ub) was transfected into HEK-293 cells together with FLAG-tagged arrestin3 or GRK2. Following a period of serum starvation lasting 4–6 h, the cells were exposed to 10 μM DA for 1–2 minutes. Cellular lysates were solubilized using a lysis buffer comprising 150 mM NaCl, 50 mM Tris pH 7.4, 1 mM EDTA, 1% Triton X-100, 10% (v/v) glycerol, 1 mM sodium orthovanadate, 1 mM sodium fluoride, 2 mM phenylmethylsulfonyl fluoride, 5 μg/mL leupeptin, 5 μg/mL aprotinin, and 10 mM N-ethylmaleimide. Immunoprecipitation was carried out using FLAG beads. The resulting immunoprecipitates underwent analysis via SDS-PAGE and subsequent blotting with antibodies targeting HA and FLAG, each diluted at a ratio of 1:1,000.
4.8. Subcellular Fractionation
Cell lysates were fractionated into cytoplasmic and nuclear fractions according to previous studies [
42,
43]. In brief, cells were incubated with buffer-1 (10 mM HEPES/KOH pH 7.8, 1.5 mM MgCl2, 10 mM KCl, 0.5 mM dithiothreitol, 0.2 mM phenylmethylsulfonyl fluoride, and 1 mM Na
3VO
4) for 20 min and centrifuged at 2,000 ×
g for 5 min. The supernatants were centrifuged for another 10 min at 15,000 ×
g following which the supernatants were saved as cytoplasmic fraction.
The pellet from the first centrifugation step was washed with buffer-1 for 15 min and centrifuged at 15,000 ×g for 10 min. The resulting pellet was incubated with buffer-2 (20 mM HEPES/KOH pH 7.8, 1.5 mM MgCl2, 420 mM NaCl, 0.2 mM EDTA, 25% glycerol, 0.5 mM dithiothreitol, 0.2 mM phenylmethylsulfonyl fluoride, and 1 mM Na3VO4) for 20 min and then centrifuged. After centrifugation at 24,000 ×g for 10 min, the supernatant was collected as a nuclear extract. Actin and lamin B1 were used as reference proteins for cytosolic and nuclear fractions, respectively.
4.9. Statistics
Data are expressed as mean ± standard deviations (SD). To control the unwanted sources of variation, some results of immunoblotting are expressed as fold of control means. The increase in the value of gel density was calculated by comparing each value with that of the mock group (usually the first lane of the gel). Comparisons between groups were performed using Student’s t-test. For the comparison of means among multiple groups, one-way ANOVA with Tukey’s post-hoc test was used. A p-value < 0.05 was considered significant.
5. Conclusions
We have unveiled the molecular mechanism underlying GRK2 ubiquitination and its functional implications. Our findings reveal that the ubiquitination of GRK2 induced by agonists in the cytosol requires the nuclear export of Mdm2, along with arrestin3 ubiquitination within the nucleus. Consequently, the ubiquitination processes of arrestin3 and GRK2 are intricately interconnected, with the cellular pathways governing arrestin3 ubiquitination also playing a vital role in GRK2 ubiquitination. Through this study, we have provided new insights into previously reported but challenging-to-understand molecular mechanisms.
Author Contributions
D.K., X.M., X.Z., X.T., S.W.: investigation, data analysis. K.M.K.: design of the experiments and writing. All authors have read and agreed to the published version of the manuscript.
Funding
This research was supported by the Basic Science Research Program through the National Research Foundation of Korea (NRF) funded by the Ministry of Education (RS-2023-00239943).
Data Availability Statement
The data that support the findings of this study are available on request from the corresponding author.
Acknowledgments
We thank the Korea Basic Science Institute for its technical support. We thank Professor Somy Yoon (College of Pharmacy, Chonnam National University) for her help with image analysis.
Conflicts of Interest
The authors declare no conflicts of interest.
References
- Benovic, J.L.; Strasser, R.H.; Caron, M.G.; Lefkowitz, R.J. Beta-adrenergic receptor kinase: identification of a novel protein kinase that phosphorylates the agonist-occupied form of the receptor. Proc Natl Acad Sci U S A 1986, 83, 2797-2801.
- Benovic, J.L., et al., Functional desensitization of the isolated beta-adrenergic receptor by the beta-adrenergic receptor kinase: potential role of an analog of the retinal protein arrestin (48-kDa protein). Proc Natl Acad Sci U S A, 1987. 84(24): p. 8879-82.
- Ferguson, S.S.; Downey, W.E., 3rd; Colapietro, A.M.; Barak, L.S.; Menard, L.; Caron, M.G. Role of beta-arrestin in mediating agonist-promoted G protein-coupled receptor internalization. Science 1996, 271, 363-366. [CrossRef]
- Goodman, O.B., Jr.; Krupnick, J.G.; Santini, F.; Gurevich, V.V.; Penn, R.B.; Gagnon, A.W.; Keen, J.H.; Benovic, J.L. Beta-arrestin acts as a clathrin adaptor in endocytosis of the beta2-adrenergic receptor. Nature 1996, 383, 447-450. [CrossRef]
- Laporte, S.A.; Oakley, R.H.; Zhang, J.; Holt, J.A.; Ferguson, S.S.; Caron, M.G.; Barak, L.S. The beta2-adrenergic receptor/betaarrestin complex recruits the clathrin adaptor AP-2 during endocytosis. Proc Natl Acad Sci U S A 1999, 96, 3712-3717.
- Shenoy, S.K.; McDonald, P.H.; Kohout, T.A.; Lefkowitz, R.J. Regulation of receptor fate by ubiquitination of activated beta 2-adrenergic receptor and beta-arrestin. Science 2001, 294, 1307-1313. [CrossRef]
- Salcedo, A.; Mayor, F., Jr.; Penela, P. Mdm2 is involved in the ubiquitination and degradation of G-protein-coupled receptor kinase 2. EMBO J 2006, 25, 4752-4762. [CrossRef]
- Zhang, X.; Min, X.; Wang, S.; Sun, N.; Kim, K.M. Mdm2-mediated ubiquitination of beta-arrestin2 in the nucleus occurs in a Gbetagamma- and clathrin-dependent manner. Biochem Pharmacol 2020, 178, 114049. [CrossRef]
- Zheng, M.; Zhang, X.; Guo, S.; Zhang, X.; Choi, H.J.; Lee, M.Y.; Kim, K.M. PKCbetaII inhibits the ubiquitination of beta-arrestin2 in an autophosphorylation-dependent manner. FEBS Lett 2015, 589, 3929-3937. [CrossRef]
- Cahilly-Snyder, L.; Yang-Feng, T.; Francke, U.; George, D.L. Molecular analysis and chromosomal mapping of amplified genes isolated from a transformed mouse 3T3 cell line. Somatic cell and molecular genetics 1987, 13, 235-244.
- Haupt, Y.; Maya, R.; Kazaz, A.; Oren, M. Mdm2 promotes the rapid degradation of p53. Nature 1997, 387, 296-299. [CrossRef]
- Honda, R.; Tanaka, H.; Yasuda, H. Oncoprotein MDM2 is a ubiquitin ligase E3 for tumor suppressor p53. FEBS letters 1997, 420, 25-27.
- Kubbutat, M.H.; Jones, S.N.; Vousden, K.H. Regulation of p53 stability by Mdm2. Nature 1997, 387, 299-303. [CrossRef]
- Chen, J.; Lin, J.; Levine, A.J. Regulation of transcription functions of the p53 tumor suppressor by the mdm-2 oncogene. Molecular medicine 1995, 1, 142-152.
- Roth, J.; Dobbelstein, M.; Freedman, D.A.; Shenk, T.; Levine, A.J. Nucleo-cytoplasmic shuttling of the hdm2 oncoprotein regulates the levels of the p53 protein via a pathway used by the human immunodeficiency virus rev protein. The EMBO journal 1998, 17, 554-564. [CrossRef]
- Vousden, K.H. p53: death star. Cell 2000, 103, 691-694.
- Wang, P.; Gao, H.; Ni, Y.; Wang, B.; Wu, Y.; Ji, L.; Qin, L.; Ma, L.; Pei, G. Beta-arrestin 2 functions as a G-protein-coupled receptor-activated regulator of oncoprotein Mdm2. J Biol Chem 2003, 278, 6363-6370. [CrossRef]
- Hoeppner, C.Z.; Cheng, N.; Ye, R.D. Identification of a nuclear localization sequence in beta-arrestin-1 and its functional implications. J Biol Chem 2012, 287, 8932-8943. [CrossRef]
- Scott, M.G.; Le Rouzic, E.; Perianin, A.; Pierotti, V.; Enslen, H.; Benichou, S.; Marullo, S.; Benmerah, A. Differential nucleocytoplasmic shuttling of beta-arrestins. Characterization of a leucine-rich nuclear export signal in beta-arrestin2. J Biol Chem 2002, 277, 37693-37701. [CrossRef]
- Kudo, N.; Wolff, B.; Sekimoto, T.; Schreiner, E.P.; Yoneda, Y.; Yanagida, M.; Horinouchi, S.; Yoshida, M. Leptomycin B inhibition of signal-mediated nuclear export by direct binding to CRM1. Exp Cell Res 1998, 242, 540-547. [CrossRef]
- Schmid, S.L. Clathrin-coated vesicle formation and protein sorting: an integrated process. Annu Rev Biochem 1997, 66, 511-548. [CrossRef]
- Guo, S.; Zhang, X.; Zheng, M.; Zhang, X.; Min, C.; Wang, Z.; Cheon, S.H.; Oak, M.H.; Nah, S.Y.; Kim, K.M. Selectivity of commonly used inhibitors of clathrin-mediated and caveolae-dependent endocytosis of G protein-coupled receptors. Biochim Biophys Acta 2015, 1848, 2101-2110. [CrossRef]
- Nobles, K.N.; Xiao, K.; Ahn, S.; Shukla, A.K.; Lam, C.M.; Rajagopal, S.; Strachan, R.T.; Huang, T.Y.; Bressler, E.A.; Hara, M.R.; et al. Distinct phosphorylation sites on the beta(2)-adrenergic receptor establish a barcode that encodes differential functions of beta-arrestin. Science signaling 2011, 4, ra51. [CrossRef]
- Cho, D.; Zheng, M.; Min, C.; Ma, L.; Kurose, H.; Park, J.H.; Kim, K.M. Agonist-induced endocytosis and receptor phosphorylation mediate resensitization of dopamine D(2) receptors. Mol Endocrinol 2010, 24, 574-586. [CrossRef]
- Gorlich, D.; Vogel, F.; Mills, A.D.; Hartmann, E.; Laskey, R.A. Distinct functions for the two importin subunits in nuclear protein import. Nature 1995, 377, 246-248. [CrossRef]
- Teh, T.; Tiganis, T.; Kobe, B. Crystallization of importin alpha, the nuclear-import receptor. Acta crystallographica. Section D, Biological crystallography 1999, 55, 561-563.
- Matsuura, Y.; Stewart, M. Structural basis for the assembly of a nuclear export complex. Nature 2004, 432, 872-877. [CrossRef]
- Lange, A.; Mills, R.E.; Lange, C.J.; Stewart, M.; Devine, S.E.; Corbett, A.H. Classical nuclear localization signals: definition, function, and interaction with importin alpha. J Biol Chem 2007, 282, 5101-5105. [CrossRef]
- Wang, P.; Wu, Y.; Ge, X.; Ma, L.; Pei, G. Subcellular localization of beta-arrestins is determined by their intact N domain and the nuclear export signal at the C terminus. J Biol Chem 2003, 278, 11648-11653. [CrossRef]
- Mangmool, S.; Haga, T.; Kobayashi, H.; Kim, K.M.; Nakata, H.; Nishida, M.; Kurose, H. Clathrin required for phosphorylation and internalization of beta2-adrenergic receptor by G protein-coupled receptor kinase 2 (GRK2). J Biol Chem 2006, 281, 31940-31949. [CrossRef]
- Pearse, B.M., Coated vesicles from pig brain: purification and biochemical characterization. J Mol Biol, 1975. 97(1): p. 93-8.
- Keen, J.H.; Willingham, M.C.; Pastan, I.H. Clathrin-coated vesicles: isolation, dissociation and factor-dependent reassociation of clathrin baskets. Cell 1979, 16, 303-312. [CrossRef]
- Min, C.; Zhang, X.; Zheng, M.; Sun, N.; Acharya, S.; Zhang, X.; Kim, K.M. Molecular Signature That Determines the Acute Tolerance of G Protein-Coupled Receptors. Biomol Ther (Seoul) 2017, 25, 239-248. [CrossRef]
- Nogues, L.; Salcedo, A.; Mayor, F., Jr.; Penela, P. Multiple scaffolding functions of {beta}-arrestins in the degradation of G protein-coupled receptor kinase 2. J Biol Chem 2011, 286, 1165-1173. [CrossRef]
- 35. Zheng, M.; Zhang, X.; Min, X.; Sun, N.; Kim, K.M. Cytoplasmic recruitment of Mdm2 as a common characteristic of G protein-coupled receptors that undergo desensitization. Biochem Biophys Res Commun 2020, 530, 181-188. [CrossRef]
- Min, X.; Sun, N.; Wang, S.; Zhang, X.; Kim, K.M. Sequestration of Gbetagamma by deubiquitinated arrestins into the nucleus as a novel desensitization mechanism of G protein-coupled receptors. Cell Commun Signal 2023, 21, 11. [CrossRef]
- Kim, K.M.; Valenzano, K.J.; Robinson, S.R.; Yao, W.D.; Barak, L.S.; Caron, M.G. Differential regulation of the dopamine D2 and D3 receptors by G protein-coupled receptor kinases and beta-arrestins. J Biol Chem 2001, 276, 37409-37414. [CrossRef]
- Zhang, X.; Min, X.; Zhu, A.; Kim, K.M. A novel molecular mechanism involved in the crosstalks between homologous and PKC-mediated heterologous regulatory pathway of dopamine D2 receptor. Biochem Pharmacol 2020, 174, 113791. [CrossRef]
- Sun, N.; Zhang, X.; Zhang, X.; Kim, K.M. The EGF receptor inhibits the signaling of dopamine D3 receptor through the phosphorylation of GRK2 on tyrosine residues. Biochem Biophys Res Commun 2017, 489, 515-522. [CrossRef]
- Min, C.; Zheng, M.; Zhang, X.; Caron, M.G.; Kim, K.M. Novel roles for beta-arrestins in the regulation of pharmacological sequestration to predict agonist-induced desensitization of dopamine D3 receptors. Br J Pharmacol 2013, 170, 1112-1129. [CrossRef]
- Adler, J.; Parmryd, I. Quantifying colocalization by correlation: the Pearson correlation coefficient is superior to the Mander's overlap coefficient. Cytometry A 2010, 77, 733-742. [CrossRef]
- Pan, W.; Jia, Y.; Wang, J.; Tao, D.; Gan, X.; Tsiokas, L.; Jing, N.; Wu, D.; Li, L. Beta-catenin regulates myogenesis by relieving I-mfa-mediated suppression of myogenic regulatory factors in P19 cells. Proc Natl Acad Sci U S A 2005, 102, 17378-17383. [CrossRef]
- Min, C.; Cho, D.I.; Kwon, K.J.; Kim, K.S.; Shin, C.Y.; Kim, K.M. Novel regulatory mechanism of canonical Wnt signaling by dopamine D2 receptor through direct interaction with beta-catenin. Mol Pharmacol 2011, 80, 68-78. [CrossRef]
|
Disclaimer/Publisher’s Note: The statements, opinions and data contained in all publications are solely those of the individual author(s) and contributor(s) and not of MDPI and/or the editor(s). MDPI and/or the editor(s) disclaim responsibility for any injury to people or property resulting from any ideas, methods, instructions or products referred to in the content. |
© 2024 by the authors. Licensee MDPI, Basel, Switzerland. This article is an open access article distributed under the terms and conditions of the Creative Commons Attribution (CC BY) license (http://creativecommons.org/licenses/by/4.0/).