1. Introduction
The CSC hypothesis was first proposed in the 1990s [
1]. Since then, it has been the subject of extensive research [
2], contributing to a better understanding of tumorigenesis and the development of more effective cancer therapies [
3].
Stem Cells (SCs) are undifferentiated, primitive cells present within an organism [
4]. When cultured in vitro, these cells often undergo epigenetic changes and exhibit dysfunctions, thus they are better described as Stem-like Cells (SLCs) [
5,
6,
7,
8,
9,
10]. In cancer research, a subset of these cells, known as CSCs, has been identified. Outside the organism, these cells are termed Cancer Stem-like Cells (CSLCs) [
2]. CSCs and CSLCs are typically malignant cells originating from epithelial tissues. Malignant cells from other germ layers have specific names; for example, in leukemia, they are called Leukemic Stem Cells (LSCs) [
1].
Initially, the CSC hypothesis was considered controversial [
11,
12,
13], but over time, and is now widely accepted [
14,
15,
16]. Among CSCs, their precursor stages have garnered significant interest and hope exploration of the mechanisms of carcinogenesis [
17]. Bhartiya et al. in 2023 described experimental results indicating that CSCs originate from transformed VSELSCs [
17]. The existence of CSCs from transformed VSELSCs, exemplified by (Very) Small Cancer Stem-Like Cells ((V)SCSLCs) and (V)SLSLCs, was confirmed a few months later that same year [
18]. It's noteworthy that VSELSCs were discovered in 2006 [
19], and like CSCs and SCs, they were initially met with skepticism [
20]. To date, many independent laboratories have confirmed the presence of VSELSCs
in vivo [
21], and recent reports about their cell culture counterparts have generated considerable interest [
18]. This uniquely addresses communication about their
in vitro analogs given the availability of experimental material in the form of cell cultures and their enrichment by efficient methods in the number of primitive stages [
18,
22,
23,
24]. There is a real possibility for comparing the healthy and malignancy most primitive stages by obtaining a minimum of 5000 (10000 preferred) cell stages for single-cell RNA sequencing analysis. These results enhance our chances of understanding the molecular mechanisms underlying malignant proliferation growth, as well as differences in cell cycle checkpoints [
25] and cell stage division types according to the Hayflick phenomenon [
26] between healthy VSELSCs and transformed (V)SCSCs as well as (V)SLSCs, and their stage analogs
in vitro.
Table 1.
Very Primitive Cellular Stages in vitro and in vivo. * Suggested names.
Table 1.
Very Primitive Cellular Stages in vitro and in vivo. * Suggested names.
Cellular Stages |
In Vivo |
In Vitro |
Stem Cells, SCs |
Stem-Like Cells, SLCs |
Cancers Stem Cells, CSCs |
Cancers Stem-Like Cells, CSLCs |
Leukemic Stem Cells, LSCs |
Leukemic Stem-Like Cells, LSLCs |
Very Small Embryonic-Like Stem Cells, VSELSCs, VSEL |
(Very) Small Embryonic-Like Stem Cells, (V)SELSCs |
*(Very) Small Cancer Stem Cells, (V)SCSCs |
(Very) Small Cancer Stem-Like Cells, (V)SCSLCs |
* Small Cancer Stem Cells, SCSCs |
Small Cancer Stem-Like Cells, SCSLCs |
* Small Leukemic Stem Cells, SLSCs |
Small Leukemic Stem-Like Cells, SLSLCs |
2. Current Knowledge
The skepticism surrounding the existence and role of VSELSCs is similar to the initial doubts about SCs and CSCs [
1,
2,
11,
12,
13]. However, identifying VSELSCs is more complex due to their size and morphological similarities to extracellular vesicles (EVs) and apoptotic bodies (ABs). These similarities make it challenging to distinguish VSELSCs from cellular debris in scatter dot plots [
27]. Despite these challenges, EVs [
28,
29,
30,
31,
32,
33,
34,
35], ABs, and VSELSCs are all significant in various biological processes. However, the ability to self-renew, grow, and proliferate is unique to VSELSCs [
36,
37,
38]. Given the morphological similarities among these structures, we provide an overview of their distinct characteristics and summarized on
Table 2.
2.1. Cytological Characteristics of Extracellular Vesicles
EVs are tiny, membrane-enclosed particles released by cells during various biological activities [
39,
40,
41,
42,
43,
44]. Their size and function vary depending on their cell of origin, which affects their environmental interactions and role in cell-to-cell communication [
39,
40,
41,
42,
43,
44]. EVs transport a variety of molecules, including proteins, lipids, nucleic acids, and signaling compounds, through interactions facilitated by their membranes. However, they are not always completely isolated from the external environment by their lipid membrane [
39,
40,
41,
42,
43,
44]. Different types of EVs include:
Ectovesicles- These protrude from the cell surface and may assist in intercellular signaling;
Exosomes- Ranging from approximately 30 to 150 nanometers in size, exosomes can be recognized by specific membrane markers and proteins;
Microvesicles- Typically measuring between 0.1 and 1 micrometer, these are released by cells in response to activation, oxidative stress, or inflammation;
Apoptosomes- Varying in size and composition based on the apoptosis stage and cell type, they are surrounded by a lipid membrane containing cellular fragments like nuclear debris or organelles [
39,
40,
41,
42,
43,
44]. The structural similarity between these EV subtypes and apoptotic bodies, which also include certain cytoplasmic elements, implies that they could be classified as a form ABs, and vice versa, ABs might be classified as EVs.
2.2. Cytological Characteristics of Apoptotic Bodies
ABs are typically small, circular or oval structures, ranging from 1 to 5 micrometers in diameter, and are formed during apoptosis, the programmed cell death process [
45,
46,
47,
48,
49,
50,
51]. During apoptosis, the cell's chromatin undergoes significant condensation [
45,
46,
47,
48,
49,
50,
51]. As the cell advances through apoptosis, it shrinks and its fragmented components are encapsulated within vesicles, which may be enveloped by a double membrane. This membrane offers some level of protection to the contents but does not ensure complete integrity [
45,
46,
47,
48,
49,
50,
51,
52]. Early-stage apoptotic bodies may not show positive staining for 7-AAD, reflecting complete isolation [
53]. Importantly, this study does not cover VSELSCs and their morphology. Furthermore, the outer membrane of ABs can display specific CD surface markers, although this is not consistent across all apoptotic bodies [
45,
46,
47,
48,
49,
50,
51]. The presence of these markers can vary depending on the cell type, stage of apoptosis, and other conditions, making it a characteristic with limited specificity.
2.3. Cytological Characteristics of Very Small Embryonic-Like Stem Cells
Human VSELSCs are small cellular structures, typically 5-7 micrometers in size, with unique embryonic characteristics such as the ability to self-renew and differentiate into various cell types [
54,
55,
56]. Advocates for VSELSCs believe these cells could play a significant role in regeneration and have therapeutic potential [
54,
55,
56]. They have been found in the peripheral blood of individuals across different age groups, from young to elderly [
57]. However, critics argue that the lack of clear definitions and universally accepted markers makes it difficult to distinguish VSELSCs from other cell types or cellular debris. While the concept of VSELSCs is promising, more detailed research is needed to understand their biological mechanisms and the accuracy of detection methods. Despite these challenges, there is a growing body of independent studies confirming the existence of VSELSCs. Cellular debris, which consists of cell fragments from disintegration, apoptosis, or other cellular activities, can be mistaken for living cells during flow cytometry analysis [
58].
2.4. Cytological Characteristics of Very Small Leukemic Stem-Like Cells
Identifying specific CD markers that uniquely target SCs, CSCs, LSCs, and their precursors is challenging [
56]. These cells might fuse with other cells, avoiding detection and potentially entering the external environment after cell death [
59,
60,
61,
62,
63,
64]. Because of their morphology, they can be mistaken for EVs and ABs in some assays, and vice versa. The involvement of EVs and ABs in cellular development is an area of intense research, as many studies indicate [
65,
66,
67,
68]. Very small cellular debris and EVs generally appear in scatter dot plot regions that are hard to count. Rarely, EVs and ABs can mimic (V)SLSLCs, being a few micrometers in size and containing DNA fragments similar to cell nuclei. Differentiating them from (V)SLSLCs is aided by a round membrane, pH environment, and mature chromatin. The use of 7-AAD dye in samples helps distinguish them on scatter dot plots from events with intact membranes. Since some EVs and ABs are similar in size and content, can display CD markers, and in early stages may not allow the entry of 7-AAD and similar dyes, distinguishing them from (very) small cell developmental stages remain challenging.
3. Proposed Cascade of Stage Transformation of Acute Myeloid Leukemia
Here, we propose models that outline potential pathways of development for (V)SELSCs (
Figure 1), shedding light on their role in acute myeloid leukemia (AML) initiation (
Figure 2 and
Figure 3). One scenario posits that only (V)SELSCs possess self-renewal capabilities, triggering asymmetric divisions upon reaching a critical cell density, resulting in the generation of SELSCs. Subsequent asymmetric divisions of SELSCs give rise to SLCs. Alternatively, another model suggests that (V)SELSCs, SELSCs, and SLCs all exhibit self-renewal abilities, leading to the formation of self-renewing SESLCs, which then differentiate into SLCs. SLCs undergo self-renewal and, upon reaching a critical cell density, initiate asymmetric divisions to produce multi- or oligopotent progenitors. In this model, each of the highly primitive precursor stages has the capability to form colonies. Their release triggers self-renewal and asymmetric division in the parental colony. These models underscore the complexity of (V)SELSCs developmental transformations and highlight the need for further research to elucidate their precise contributions to cancer or leukemia initiation and progression (
Figure 1). Similarly, examples of AML origin from (V)SELSCs outline various scenarios of leukemia initiation, emphasizing the interplay between (V)SELSCs, (V)SLSLCs, SELSCs, SLCs, and LSLCs at different stages (
Figure 2). The inclusion of fusion events in these models further underscores the intricate nature of AML pathogenesis and warrants exploration in future studies to comprehensively understand the mechanisms driving leukemia development (
Figure 3).
4. Benefits from Future Research Findings
4.1. Preclinical and Clinical Study
In preclinical research, considering (V)SLSLCs can assist in identifying the most effective potential chemotherapeutic agent by testing IC50 values for cell viability [
22]. These findings are relevant in both preclinical and clinical trials (if their in vivo counterparts are included), aiding in the selection of the most efficient and least toxic drug. Moreover, such studies may enhance our understanding of metabolic pathways applicable in therapy.
4.2. Potential Clinical Applications
Targeted therapies: Identifying specific markers and mechanisms that lead to the transformation of VSELSCs and (V)SELSCs into cancer cells could enable the development of targeted therapies. Such therapies could precisely attack cancer cells at early stages of their development, potentially being more effective than current treatments.
Early diagnosis: Charatreristics of VSELSCs and (V)SELSCs as precursors to CSCs and LSCs could lead to the development of new biomarkers for earlier cancer detection. Early diagnosis is crucial for effective treatment and improved patient outcomes. New therapeutic targets: Identifying and characterizing VSELSCs as precursors to CSCs and LSCs opens new therapeutic opportunities. This research could lead to the development of therapies that are more effective in eliminating cancers at their earliest stages.
Tissue regeneration: Since VSELSCs have the ability to differentiate into various cell types, they might be used in regenerative medicine to repair of damaged tissues. This application could be relevant not only for cancer treatment but also for other conditions requiring tissue regeneration.
Resistance to treatment: Research on VSELSCs and (V)SELSCs could help elucidate the mechanisms by which cancer cells resist treatment. Understanding how these cells evade therapies could lead to new strategies to overcome this resistance.
4.3. Extend the Konwledge Abaout Cancerogenesis - Epigenetic Analysis of Cells
Filling knowledge gaps: Previous research on CSCs has primarily focused on more developed cancer cell stages. Studying VSELSCs, which are earlier precursors (quite possibly the most primitive cell stage in young, middle-aged and aged human organisms [
73]), can provide new insights into the initial stages of carcinogenesis, which are currently poorly understood. Comparison of healthy and cancerous cells: Research on the differences between healthy VSELSCs and their cancerous counterparts (V)SCSLCs and (V)SLSLCs can reveal key molecular and epigenetic mechanisms responsible for cancer transformation. This can aid in identifying new diagnostic markers and therapeutic targets.
Gene regulation mechanisms: Epigenetics studies changes in gene expression that are not caused by changes in the DNA sequence. Epigenetic analysis of VSELSCs can reveal which epigenetic changes lead to their transformation into cancer cells. Marker identification: Epigenetic markers can be used to identify early stages of cancer development. For example, DNA methylation and histone modifications specific to VSELSCs could serve as biomarkers for early diagnosis. Therapy development: Understanding the epigenetic mechanisms regulating VSELSCs function could lead to the development of new epigenetic therapies that can reverse or inhibit cancer transformation.
4.4. Interactions Between Most Primitive Cancer and Healthly Precursors with the Cellular Microenvironment
Impact of the microenvironment: The cellular microenvironment plays a key role in regulating the growth, survival, and differentiation of cancer cells. Understanding how VSELSCs interact with surrounding cells can reveal mechanisms that promote or inhibit carcinogenesis.
Adaptation capability: Studying interactions between these cells and their microenvironment can reveal how cells adapt to different conditions. This is important for understanding how cancers develop resistance to treatment and how this phenomenon can be overcome.
New therapeutic strategies: Interactions between cells and the microenvironment can be targeted by new therapies. For example, disrupting signals between VSELSCs or (V)SELSCs and surrounding cells could be an effective strategy in cancer treatment.
5. Conclusions
Drawing an analogy to developmental stage-dependent transformations, consider the study of silkworm (Bombyx mori) instars under controlled conditions that shorten their developmental cycle. Even with this accelerated development, silkworms still progress through the stages of egg, caterpillar, pupa, and moth. However, the duration of each stage is reduced; for example, caterpillars can transition into pupae without undergoing the complete range of instars [
74,
75,
76,
77,
78].
If VSELSCs serve as precursors to SCs and CSCs, it is highly probable that analogous precursor cell stages would be present in continuously growing cell cultures, despite the accumulation of various changes and alterations.
In cancer cell cultures in vitro, healthy stages of (very) small precursor stages are rather absent. However, homologous stages of VSELSCs are observed instead. This suggests that this developmental stage is highly likely to be cancerous. If this is the case, it is equally probable that these stages may also be diseased in vivo.
Given the availability of experimental material in the form of cell cultures, their increased proliferation rate compared to healthy cells, and the efficient method of enriching the cultures with primitive stages, there is a real possibility of obtaining enough cells for single-cell RNA sequencing (transcriptomics). This analysis requires a minimum of 5000 to 10000 cells to initiate the reaction. These results enhance our chances of understanding the molecular mechanisms underlying malignant proliferation growth, as well as differences in cell divisions (the Hayflick phenomenon) between healthy VSELSCs and (V)SELSCs and transformed (V)SCSCs and (V)SCSLCs as well as (V)SLSCs and (V)SLSLCs.
Author Contributions
Conceptualization, J.J.L, J.JB. and A.H.; investigation, J.J.L, J.JB. and A.H; resources, J.JL.; writing—original draft preparation, J.J.L, J.JB. and A.H; visualization, J.J.L,.; supervision, J.J.L; project administration, J.J.L and J.J.B; funding acquisition, J.J.L.; All authors have read and agreed to the published version of the manuscript.
Funding
The APC was funded by Cancer (MDPI, Basel).
Institutional Review Board Statement
Not applicable.
Informed Consent Statement
Not applicable.
Data Availability Statement
We encourage all authors of articles published in MDPI journals to share their research data. In this section, please provide details regarding where data supporting reported results can be found, including links to publicly archived datasets analyzed or generated during the study. Where no new data were created, or where data is unavailable due to privacy or ethical restrictions, a statement is still required. Suggested Data Availability Statements are available in section “MDPI Research Data Policies” at
https://www.mdpi.com/ethics.
Acknowledgments
We thank G. Grabe for critical reading of the manuscript.
Conflicts of Interest
The authors declare no conflicts of interest.
References
- Bonnet, D.; Dick, J.E. Human acute myeloid leukemia is organized as a hierarchy that originates from a primitive hematopoietic cell. Nat. Med. 1997, 3, 730–737. [Google Scholar] [CrossRef]
- Kondo, T.; Setoguchi, T.; Taga, T. Persistence of a small subpopulation of cancer stem-like cells in the C6 glioma cell line. Proc. Natl. Acad. Sci. 2004, 101, 781–786. [Google Scholar] [CrossRef] [PubMed]
- Clarke, M.F.; Dick, J.E.; Dirks, P.B.; Eaves, C.J.; Jamieson, C.H.M.; Jones, D.L.; Visvader, J.; Weissman, I.L.; Wahl, G.M. Cancer Stem Cells—Perspectives on Current Status and Future Directions: AACR Workshop on Cancer Stem Cells. Cancer Res. 2006, 66, 9339–9344. [Google Scholar] [CrossRef] [PubMed]
- Clarke, M.F.; Fuller, M. Stem Cells and Cancer: Two Faces of Eve. Cell 2006, 124, 1111–1115. [Google Scholar] [CrossRef]
- Nestor, C.E.; Ottaviano, R.; Reinhardt, D.; Cruickshanks, H.A.; Mjoseng, H.K.; McPherson, R.C.; Lentini, A.; Thomson, J.P.; Dunican, D.S.; Pennings, S.; et al. Rapid reprogramming of epigenetic and transcriptional profiles in mammalian culture systems. Genome Biol. 2015, 16, 1–17. [Google Scholar] [CrossRef]
- Weissbein, U.; Plotnik, O.; Vershkov, D.; Benvenisty, N. Culture-induced recurrent epigenetic aberrations in human pluripotent stem cells. PLOS Genet. 2017, 13, e1006979. [Google Scholar] [CrossRef]
- Bar, S.; Benvenisty, N. Epigenetic aberrations in human pluripotent stem cells. EMBO J. 2019, 38. [Google Scholar] [CrossRef]
- Tognon, C.E.; Sears, R.C.; Mills, G.B.; Gray, J.W.; Tyner, J.W. Ex Vivo Analysis of Primary Tumor Specimens for Evaluation of Cancer Therapeutics. Annu. Rev. Cancer Biol. 2021, 5, 39–57. [Google Scholar] [CrossRef]
- Liu, Z.; Leung, D.; Thrush, K.; Zhao, W.; Ratliff, S.; Tanaka, T.; Schmitz, L.L.; Smith, J.A.; Ferrucci, L.; Levine, M.E. Underlying features of epigenetic aging clocks in vivo and in vitro. Aging Cell 2020, 19. [Google Scholar] [CrossRef]
- Kabacik, S.; Lowe, D.; Fransen, L.; Leonard, M.; Ang, S.-L.; Whiteman, C.; Corsi, S.; Cohen, H.; Felton, S.; Bali, R.; et al. The relationship between epigenetic age and the hallmarks of aging in human cells. Nat. Aging 2022, 2, 484–493. [Google Scholar] [CrossRef]
- Smith, J.K.; Johnson, L.M. Do non-tumorigenic cells have stem cell potency? Nat. Med. 1999, 5, 261–262. [Google Scholar] [CrossRef]
- Coleman, M.L.; Sahai, E.A.; Yeo, M.; Bosch, M.; Dewar, A.; Olson, M.F. Membrane blebbing during apoptosis results from caspase-mediated activation of ROCK I. Nat. Cell Biol. 2001, 3, 339–345. [Google Scholar] [CrossRef]
- Alison, M.R.; Lim, S.M.L.; Nicholson, L.J. Cancer Stem Cells: Fact or Fiction? Biochem Biophys Res Commun. 2002, 298, 151–156. [Google Scholar] [CrossRef]
- Liu, Q.; Guo, Z.; Li, G.; Zhang, Y.; Liu, X.; Li, B.; Wang, J.; Li, X. Cancer stem cells and their niche in cancer progression and therapy. Cancer Cell Int. 2023, 23, 1–12. [Google Scholar] [CrossRef] [PubMed]
- Najafi, M.; Farhood, B.; Mortezaee, K. Cancer stem cells (CSCs) in cancer progression and therapy. J. Cell. Physiol. 2018, 234, 9698–9710. [Google Scholar] [CrossRef]
- Batlle, E.; Clevers, H. Cancer stem cells revisited. Nat. Med. 2017, 23, 1124–1134. [Google Scholar] [CrossRef]
- Bhartiya, D.; Sharma, N.; Dutta, S.; Kumar, P.; Tripathi, A.; Tripathi, A. Very Small Embryonic-Like Stem Cells Transform Into Cancer Stem Cells and Are Novel Candidates for Detecting/Monitoring Cancer by a Simple Blood Test. STEM CELLS 2023, 41, 310–318. [Google Scholar] [CrossRef]
- Lica, J.J.; Pradhan, B. (Very) Small Stem-like Cells in Human Cell Cultures. Cancers 2023, 15, 5520. [Google Scholar] [CrossRef] [PubMed]
- Ratajczak, M.Z.; Machalinski, B.; Wojakowski, W.; Ratajczak, J.; Kucia, M.; Liu, R. A hypothesis for an embryonic origin of pluripotent Oct-4+ stem cells in adult bone marrow and other tissues. Leukemia 2006, 20, 1155–1158. [Google Scholar] [CrossRef] [PubMed]
- De Coppi, P.; et al. Lack of evidence for pluripotency in human amniotic fluid stem cells. Stem Cells. 2007, 25, 1734–5. [Google Scholar] [CrossRef]
- Ratajczak, M.Z.; Ratajczak, J.; Kucia, M. Very Small Embryonic-Like Stem Cells (VSELs). Circ. Res. 2019, 124, 208–210. [Google Scholar] [CrossRef] [PubMed]
- Lica, J.J.; Wieczór, M.; Grabe, G.J.; Heldt, M.; Jancz, M.; Misiak, M.; Gucwa, K.; Brankiewicz, W.; Maciejewska, N.; Stupak, A.; et al. Effective Drug Concentration and Selectivity Depends on Fraction of Primitive Cells. Int. J. Mol. Sci. 2021, 22, 4931. [Google Scholar] [CrossRef] [PubMed]
- Lica, J.J.; Grabe, G.J.; Heldt, M.; Misiak, M.; Bloch, P.; Serocki, M.; Switalska, M.; Wietrzyk, J.; Baginski, M.; Hellmann, A.; et al. Cell Density-Dependent Cytological Stage Profile and Its Application for a Screen of Cytostatic Agents Active Toward Leukemic Stem Cells. Stem Cells Dev. 2018, 27, 488–513. [Google Scholar] [CrossRef] [PubMed]
- Csaszar, E.; Kirouac, D.C.; Yu, M.; Wang, W.; Qiao, W.; Cooke, M.P.; Boitano, A.E.; Ito, C.; Zandstra, P.W. Rapid Expansion of Human Hematopoietic Stem Cells by Automated Control of Inhibitory Feedback Signaling. Cell Stem Cell 2012, 10, 218–229. [Google Scholar] [CrossRef] [PubMed]
- Skladanowski, A.; Bozko, P.; Sabisz, M. DNA Structure and Integrity Checkpoints during the Cell Cycle and Their Role in Drug Targeting and Sensitivity of Tumor Cells to Anticancer Treatment. Chem. Rev. 2009, 109, 2951–2973. [Google Scholar] [CrossRef]
- Hayflick, L. The Limited In Vitro Life of Human Diploid Cell Strains. Exp Cell Res. 1965, Exp Cell Res., 614–636. [Google Scholar] [CrossRef]
- Kucia, M.; Reca, R.; Campbell, F.R.; et al. A population of very small embryonic-like (VSEL) CXCR4+ SSEA-1+ Oct-4+ stem cells identified in adult bone marrow. Leukemia 2006, 20, 888–891. [Google Scholar] [CrossRef]
- Savina, A.; Furlán, M.; Vidal, M.; Colombo, M.I. Exosome Release Is Regulated by a Calcium-dependent Mechanism in K562 Cells. J. Biol. Chem. 2003, 278, 20083–20090. [Google Scholar] [CrossRef] [PubMed]
- Zomer, A.; Maynard, C.; Verweij, F.J.; Kamermans, A.; Schäfer, R.; Beerling, E.; Schiffelers, R.M.; de Wit, E.; Berenguer, J.; Ellenbroek, S.I.J.; et al. In Vivo Imaging Reveals Extracellular Vesicle-Mediated Phenocopying of Metastatic Behavior. Cell 2015, 161, 1046–1057. [Google Scholar] [CrossRef]
- Zomer, A.; Maynard, C.; Verweij, F.J.; Kamermans, A.; Schafer, R.; Doeppner, T.R.; Herz, J.; Görgens, A.; Schlechter, J.; Ludwig, A.K.; Radtke, S.; et al. Extracellular vesicles improve post-stroke neuroregeneration and prevent postischemic immunosuppression. Stem Cells Transl Med. 2015, 4, 1131–1143. [Google Scholar] [CrossRef]
- Yáñez-Mó, M.; Siljander, P.R.-M.; Andreu, Z.; Bedina Zavec, A.; Borràs, F.E.; Buzas, E.I.; Buzas, K.; Casal, E.; Cappello, F.; Carvalho, J.; et al. Biological properties of extracellular vesicles and their physiological functions. J. Extracell. Vesicles 2015, 4, 27066. [Google Scholar] [CrossRef] [PubMed]
- Machtinger, R.; Laurent, L.C.; Baccarelli, A.A. Extracellular Vesicles: Roles in Gamete Maturation, Fertilization and Embryo Implantation. Hum. Reprod. Update 2016, 22, 182–193. [Google Scholar] [CrossRef] [PubMed]
- Tkach, M.; Théry, C. Communication by Extracellular Vesicles: Where We Are and Where We Need to Go. Cell 2016, 164, 1226–1232. [Google Scholar] [CrossRef] [PubMed]
- Bobis-Wozowicz, S.; Kmiotek, K.; Sekula, M.; et al. Diverse impact of xenofree conditions on biological and regenerative properties of hUC-MSCs and their extracellular vesicles. J Mol Med. 2017, 95, 205–220. [Google Scholar] [CrossRef] [PubMed]
- Kalluri, R.; LeBleu, V.S. The biology, function, and biomedical applications of exosomes. Science 2020, 367, eaau6977. [Google Scholar] [CrossRef] [PubMed]
- Ratajczak, M.Z.; Kucia, M.; Reca, R.; et al. Evidence That Very Small Embryonic-Like Stem Cells Are Mobilized into Peripheral Blood. Stem Cells 2008, 26, 2083–2092. [Google Scholar] [CrossRef] [PubMed]
- Prada, I.L.S.; Lima, C.A.; Souza, A.F.P.; Maganhin, C.C.; Cury, S.S.; Reis, A.B. Embryonic-like stem cells derived from ovarian teratomas and their spontaneous differentiation into oocyte-like cells. Sci. Rep. 2020, 10, 1–11. [Google Scholar] [CrossRef]
- Domingues, A.; Rossi, E.; Bujko, K.; Detriche, G.; Richez, U.; Blandinieres, A.; Kucia, M.; Ratajczak, J.; Smadja, D.M.; Ratajczak, M.Z. Human CD34+ very small embryonic-like stem cells can give rise to endothelial colony-forming cells with a multistep differentiation strategy using UM171 and nicotinamide acid. Leukemia 2022, 36, 1440–1443. [Google Scholar] [CrossRef] [PubMed]
- Kowal, J.; Arras, G.; Colombo, M.; Jouve, M.; Morath, J.P.; Primdal-Bengtson, B.; Dingli, F.; Loew, D.; Tkach, M.; Théry, C. Proteomic comparison defines novel markers to characterize heterogeneous populations of extracellular vesicle subtypes. Proc. Natl. Acad. Sci. USA 2016, 113, E968–E977. [Google Scholar] [CrossRef]
- Théry, C.; Witwer, K.W.; Aikawa, E.; Alcaraz, M.J.; Anderson, J.D.; Andriantsitohaina, R.; Antoniou, A.; Arab, T.; Archer, F.; Atkin-Smith, G.K.; et al. Minimal information for studies of extracellular vesicles 2018 (MISEV2018): A position statement of the International Society for Extracellular Vesicles and update of the MISEV2014 guidelines. J. Extracell. Vesicles 2018, 7, 1535750. [Google Scholar] [CrossRef]
- Van Niel, G.; D’Angelo, G.; Raposo, G. Shedding light on the cell biology of extracellular vesicles. Nat. Rev. Mol. Cell Biol. 2018, 19, 213–228. [Google Scholar] [CrossRef]
- Muhsin-Sharafaldine, M.-R.; McLellan, A.D. Tumor-Derived Apoptotic Vesicles: With Death They Do Part. Front. Immunol. 2018, 9, 957. [Google Scholar] [CrossRef] [PubMed]
- Smith, J.; Lötvall, J. Isolation and characterization of extracellular vesicle subpopulations from tissues. Nat Protoc. 2019, 14, 6–18. [Google Scholar] [CrossRef]
- Jeppesen, D.K.; Fenix, A.M.; Franklin, J.L.; Higginbotham, J.N.; Zhang, Q.; Zimmerman, L.J.; Liebler, D.C.; Ping, J.; Liu, Q.; Evans, R.; et al. Reassessment of Exosome Composition. Cell 2019, 177, 428–445. [Google Scholar] [CrossRef]
- Kerr, J.F.R.; Wyllie, A.H.; Currie, A.R. Apoptosis: A Basic Biological Phenomenon with Wideranging Implications in Tissue Kinetics. Br. J. Cancer 1972, 26, 239–257. [Google Scholar] [CrossRef] [PubMed]
- Coleman, M.L.; Sahai, E.A.; Yeo, M.; Bosch, M.; Dewar, A.; Olson, M.F. Membrane blebbing during apoptosis results from caspase-mediated activation of ROCK I. Nat. Cell Biol. 2001, 3, 339–345. [Google Scholar] [CrossRef] [PubMed]
- Zhang, Y.; Chen, X.; Gueydan, C.; et al. Plasma membrane changes during programmed cell deaths. Cell Res. 2018, 28, 9–21. [Google Scholar] [CrossRef]
- Dou, G.; Tian, R.; Liu, X.; Yuan, P.; Ye, Q.; Liu, J.; Liu, S.; Zhou, J.; Deng, Z.; Chen, X.; et al. Chimeric apoptotic bodies functionalized with natural membrane and modular delivery system for inflammation modulation. Sci. Adv. 2020, 6, eaba2987. [Google Scholar] [CrossRef]
- Serrano-Heras, G.; Díaz-Maroto, I.; Castro-Robles, B.; Carrión, B.; Perona-Moratalla, A.B.; Gracia, J.; Arteaga, S.; Hernández-Fernández, F.; García-García, J.; Ayo-Martín, O.; et al. Isolation and Quantification of Blood Apoptotic Bodies, a Non-invasive Tool to Evaluate Apoptosis in Patients with Ischemic Stroke and Neurodegenerative Diseases. Biol. Proced. Online 2020, 22, 1–17. [Google Scholar] [CrossRef]
- Aoki, K.; Satoi, S.; Harada, S.; Uchida, S.; Iwasa, Y.; Ikenouchi, J. Coordinated changes in cell membrane and cytoplasm during maturation of apoptotic bleb. Mol. Biol. Cell 2020, 31, 833–844. [Google Scholar] [CrossRef]
- Lecoeur, H.; Ledru, E.; Prévost, M.-C.; Gougeon, M.-L. Strategies for phenotyping apoptotic peripheral human lymphocytes comparing ISNT, annexin-V and 7-AAD cytofluorometric staining methods. J. Immunol. Methods 1997, 209, 111–123. [Google Scholar] [CrossRef] [PubMed]
- Zembruski, N.C.; Stache, V.; Haefeli, W.E.; Weiss, J. 7-Aminoactinomycin D for apoptosis staining in flow cytometry. Anal. Biochem. 2012, 429, 79–81. [Google Scholar] [CrossRef]
- Montgomery, S.T.; Stick, S.M.; Kicic, A. An adapted novel flow cytometry methodology to delineate types of cell death in airway epithelial cells. J. Biol. Methods 2020, 7, e139–e139. [Google Scholar] [CrossRef] [PubMed]
- Smith, J.R.; Pochampally, R.; Perry, A.; Hsu, S.C.; Prockop, D.J. Isolation of very small embryonic-like stem cells from murine and human bone marrow. Blood 2008, 111, 5494–5502. [Google Scholar] [CrossRef]
- Sovalat, H.; Scrofani, M.; Eidenschenk, A.; et al. Identification and isolation from either adult human bone marrow or G-CSF-mobilized peripheral blood of CD34(+)/CD133(+)/CXCR4(+)/ Lin(-)CD45(-) cells, featuring morphological, molecular, and phenotypic characteristics of very small embryonic-like (VSEL) stem cells. Exp Hematol. 2011, 39, 495–505. [Google Scholar] [PubMed]
- Rix, B.; Maduro, A.H.; Bridge, K.S.; Grey, W. Markers for human haematopoietic stem cells: The disconnect between an identification marker and its function. Front. Physiol. 2022, 13, 1009160. [Google Scholar] [CrossRef]
- Sovalat, H.; Scrofani, M.; Eidenschenk, A.; Hénon, P. Human Very Small Embryonic-Like Stem Cells Are Present in Normal Peripheral Blood of Young, Middle-Aged, and Aged Subjects. Stem Cells Int. 2016, 2016, 1–8. [Google Scholar] [CrossRef]
- Denzer, K.; Kleijmeer, M.J.; Heijnen, H.F.G.; Stoorvogel, W.; Geuze, H.J. Exosome: From internal vesicle of the multivesicular body to intercellular signaling device. J. Cell Sci. 2000, 113, 3365–3374. [Google Scholar] [CrossRef]
- Palovuori, R.; Myrsky, E.; Eskelinen, S. Membrane Potential and Endocytic Activity Control Disintegration of Cell-Cell Adhesion and Cell Fusion in Vinculin-Injected MDBK Cells. Journal of Cellular Physiology 2004, 200, 417–427. [Google Scholar] [CrossRef]
- Erenpreisa, J.; Salmina, K.; Huna, A.; Jackson, T.R.; Vazquez-Martin, A.; Cragg, M.S. The "Virgin Birth", Polyploidy, and the Origin of Cancer. Oncoscience 2014, 2, 3–14. [Google Scholar] [CrossRef]
- Platt, J.L.; Cascalho, M. Non-Canonical B Cell Functions in Transplantation. Human Immunology 2019, 80, 363–377. [Google Scholar] [CrossRef]
- Alhaddad, L.; Osipov, A.N.; Leonov, S. The Molecular and Cellular Strategies of Glioblastoma and Non-Small-Cell Lung Cancer Cells Conferring Radioresistance. International Journal of Molecular Sciences 2022, 23, 13577. [Google Scholar] [CrossRef]
- Vainshelbaum, N.M.; Giuliani, A.; Salmina, K.; Pjanova, D.; Erenpreisa, J. The Transcriptome and Proteome Networks of Malignant Tumours Reveal Atavistic Attractors of Polyploidy-Related Asexual Reproduction. International Journal of Molecular Sciences 2022, 23, 14930. [Google Scholar] [CrossRef]
- Vinogradov, A.E.; Anatskaya, O.V. Systemic Alterations of Cancer Cells and Their Boost by Polyploidization: Unicellular Attractor (UCA) Model. International Journal of Molecular Sciences 2023, 24, 6196. [Google Scholar] [CrossRef]
- Phan, T.K.; Ozkocak, D.C.; Poon, I.K.H. Unleashing the Therapeutic Potential of Apoptotic Bodies. Biochemical Society Transactions 2020, 48, 2079–2088. [Google Scholar] [CrossRef]
- Migneault, F.; Dieudé, M.; Turgeon, J.; Beillevaire, D.; Hardy, M.P.; Brodeur, A. , Thibodeau, N., Perreault, C.; Hébert, M.J. Apoptotic Exosome-like Vesicles Regulate Endothelial Gene Expression, Inflammatory Signaling, and Function Through the NF-κB Signaling Pathway. Scientific Reports 2020, 10, 12562. [Google Scholar] [CrossRef]
- Beltraminelli, T.; Perez, C.R.; De Palma, M. Disentangling the Complexity of Tumor-Derived Extracellular Vesicles. Cell Reports 2021, 35, 108960. [Google Scholar] [CrossRef]
- Gregory, C.D.; Rimmer, M.P. Extracellular Vesicles Arising from Apoptosis: Forms, Functions, and Applications. The Journal of Pathology Advance online publication. 2023. [Google Scholar] [CrossRef]
- Jones, P.A.; Baylin, S.B. The Epigenomics of Cancer. Cell 2007, 128, 683–692. [Google Scholar] [CrossRef] [PubMed]
- Polyak, K.; Weinberg, R.A. Transitions between epithelial and mesenchymal states: Acquisition of malignant and stem cell traits. Nat. Rev. Cancer 2009, 9, 265–273. [Google Scholar] [CrossRef] [PubMed]
- Shin, D.M.; Liu, R.; Kucia, M.; Ratajczak, J.; Ratajczak, M.Z. Molecular characterization of isolated and expanded very small embryonic/epiblast-like stem cells: A population of adult pluripotent stem cells distinct from embryonic stem cells. Leukemia 2010, 24, 1450–1461. [Google Scholar] [CrossRef]
- Easwaran, H.; Tsai, H.-C.; Baylin, S.B. Cancer Epigenetics: Tumor Heterogeneity, Plasticity of Stem-like States, and Drug Resistance. Mol. Cell 2014, 54, 716–727. [Google Scholar] [CrossRef]
- Sovalat, H.; Scrofani, M.; Eidenschenk, A.; Hénon, P. Human Very Small Embryonic-Like Stem Cells Are Present in Normal Peripheral Blood of Young, Middle-Aged, and Aged Subjects. Stem Cells Int. 2016, 2016, 1–8. [Google Scholar] [CrossRef]
- Hirase, S.; Kusakabe, T.; Oshima, I. Shortened metamorphosis in the silkworm, Bombyx mori (Lepidoptera: Bombycidae). Appl. Èntomol. Zool. 2002, 37, 79–84. [Google Scholar] [CrossRef]
- Tomita, M.; Munetsuna, H.; Sato, T.; Adachi, T.; Hino, R.; Hayashi, M.; Shimizu, K.; Nakazawa, Y.; Takahashi, M.; Nakajima, Y. Transgenic silkworms produce recombinant human type III procollagen in cocoons. Nat Biotechnol. 2003, 21, 52–56. [Google Scholar] [CrossRef]
- Goldsmith, M.R.; Shimada, T.; Abe, H. The genetics and genomics of the silkworm, Bombyx mori. Annu. Rev. Èntomol. 2005, 50, 71–100. [Google Scholar] [CrossRef]
- Pacheco, S.E.; Guerreiro, R.; Mendes, A.M.; Martins, N.E. Shortened life-cycle strains of Bombyx mori: The impact of insecticides. Sci Rep. 2014, 4, 6386. [Google Scholar] [CrossRef]
- Khan, A.A.; Munir, A.; Jilani, G. Shortening the Life-Cycle of the Silk Worm (Bombyx mori L.). J Entomol Soc Pak. 2016, 38, 51–56. [Google Scholar]
Figure 1.
Proposed examples of the stage development of (V)SELSCs. (a) In this model, only (V)SELSCs can self-renew. Once they reach a critical cell density, they start asymmetric divisions, which produce SELSCs. The asymmetric divisions of SELSCs lead to the formation of SLC); (b) In this model, (V)SELSCs, SELSCs, and SLCs all can self-renew. After reaching a critical density through self-renewal, (V)SELSCs initiate asymmetric division to form SESLCs. Self-renewing SESLCs, upon reaching critical cell density, start asymmetric divisions to produce SLCs. Similarly, SLCs also self-renew and, upon reaching a critical cell density, initiate asymmetric divisions to produce multi- or oligopotent progenitors; (c) This model assumes that (V)SELSCs and SELSCs divide asymmetrically, and replication of these cells might occur during cells fusion; In the proposed cell fusion models, we assumed that all cells within a given stage, as well as the first and last cell stages, are capable of fusion - middle stage was not used in this model, however its self-renewal is also probable; It is possible that SESLCs might be a form of (V)SELSCs undergoing self-renewal or asymmetric divisions, and there may be other intermediate stages not included in the presented examples; It is also not excluded that very small or small precursor stages represent a certain form of "dormancy," and their activation into life processes occurs after fusion with a functioning cell.
Figure 1.
Proposed examples of the stage development of (V)SELSCs. (a) In this model, only (V)SELSCs can self-renew. Once they reach a critical cell density, they start asymmetric divisions, which produce SELSCs. The asymmetric divisions of SELSCs lead to the formation of SLC); (b) In this model, (V)SELSCs, SELSCs, and SLCs all can self-renew. After reaching a critical density through self-renewal, (V)SELSCs initiate asymmetric division to form SESLCs. Self-renewing SESLCs, upon reaching critical cell density, start asymmetric divisions to produce SLCs. Similarly, SLCs also self-renew and, upon reaching a critical cell density, initiate asymmetric divisions to produce multi- or oligopotent progenitors; (c) This model assumes that (V)SELSCs and SELSCs divide asymmetrically, and replication of these cells might occur during cells fusion; In the proposed cell fusion models, we assumed that all cells within a given stage, as well as the first and last cell stages, are capable of fusion - middle stage was not used in this model, however its self-renewal is also probable; It is possible that SESLCs might be a form of (V)SELSCs undergoing self-renewal or asymmetric divisions, and there may be other intermediate stages not included in the presented examples; It is also not excluded that very small or small precursor stages represent a certain form of "dormancy," and their activation into life processes occurs after fusion with a functioning cell.
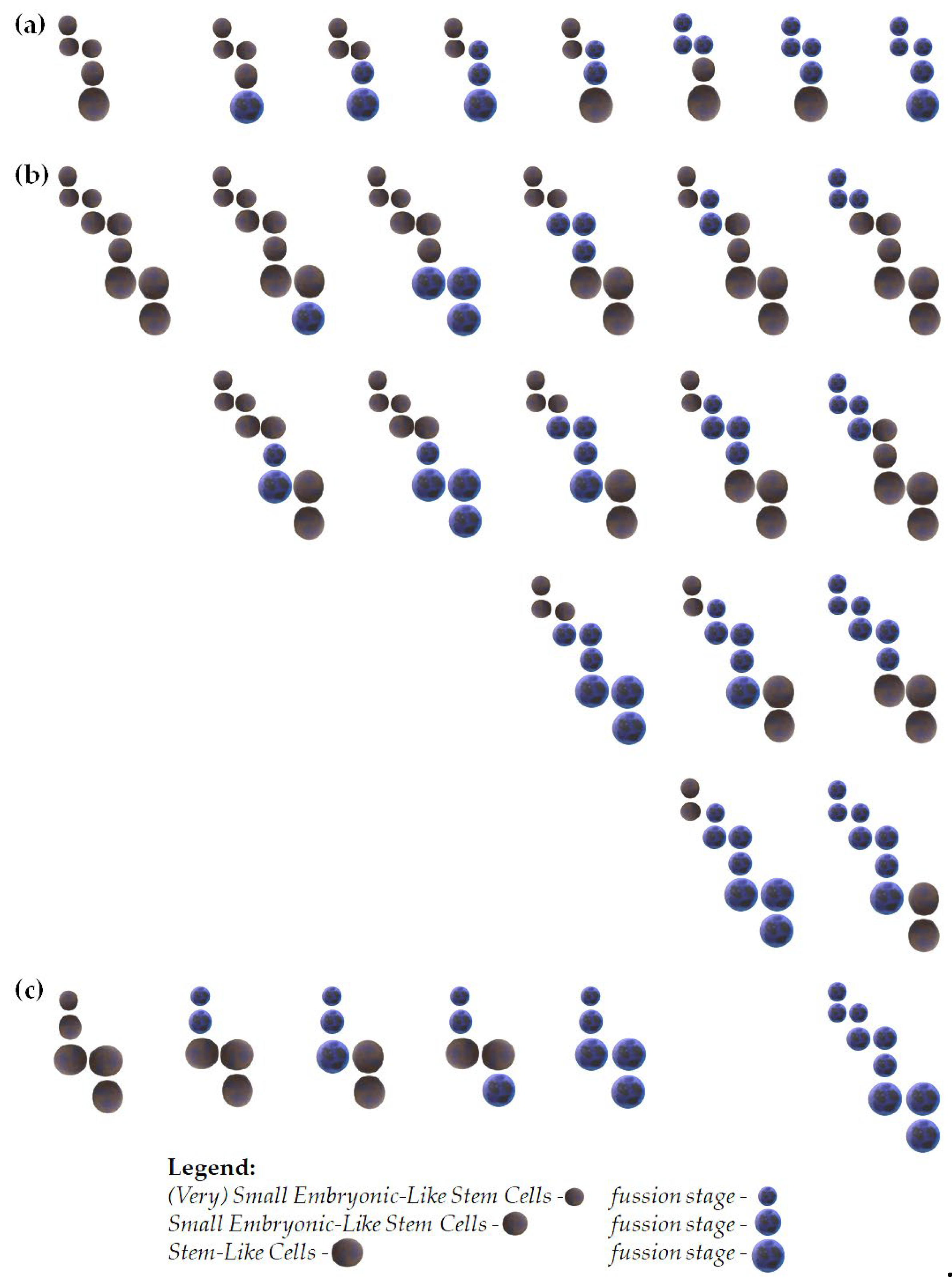
Figure 2.
Proposed examples of acute myeloid leukemia origin from (V)SELSCs. (a) Leukemia initiation through the accumulation of changes in pathogenic/pathological cells in a model where the self-renewing stage are (V)SELSCs; (b) Leukemia initiation through the accumulation of changes in pathogenic/pathological cells in a model where the self-renewing stages are (V)SELSCs and (V)SLSLCs, SELSCs and SLSLCs as well as SLCs and LSLCs; (c) Examples of leukemia initiation in a model where the self-renewing stages are (V)SELSCs and (V)SLSLCs, SELSCs and SLSLCs, as well as LSLCs; Leukemia initiation through the accumulation of changes in pathogenic/pathological cells in a model where the self-renewing stage are (V)SELSCs; This model assumes that (V)SELSCs and SELSCs divide asymmetrically, and replication of these cells might occur during LSLCs self-renewing; It is possible that SELSCs and/or LSLCs might be a form of (V)SELSCs and/or (V)SLSLCs undergoing self-renewal or asymmetric divisions, and there may be other intermediate stages not included in the presented examples; It is also not excluded that very small or small precursor stages represent a certain form of "dormancy," and their activation into life processes occurs after fusion with a functioning cell.
Figure 2.
Proposed examples of acute myeloid leukemia origin from (V)SELSCs. (a) Leukemia initiation through the accumulation of changes in pathogenic/pathological cells in a model where the self-renewing stage are (V)SELSCs; (b) Leukemia initiation through the accumulation of changes in pathogenic/pathological cells in a model where the self-renewing stages are (V)SELSCs and (V)SLSLCs, SELSCs and SLSLCs as well as SLCs and LSLCs; (c) Examples of leukemia initiation in a model where the self-renewing stages are (V)SELSCs and (V)SLSLCs, SELSCs and SLSLCs, as well as LSLCs; Leukemia initiation through the accumulation of changes in pathogenic/pathological cells in a model where the self-renewing stage are (V)SELSCs; This model assumes that (V)SELSCs and SELSCs divide asymmetrically, and replication of these cells might occur during LSLCs self-renewing; It is possible that SELSCs and/or LSLCs might be a form of (V)SELSCs and/or (V)SLSLCs undergoing self-renewal or asymmetric divisions, and there may be other intermediate stages not included in the presented examples; It is also not excluded that very small or small precursor stages represent a certain form of "dormancy," and their activation into life processes occurs after fusion with a functioning cell.
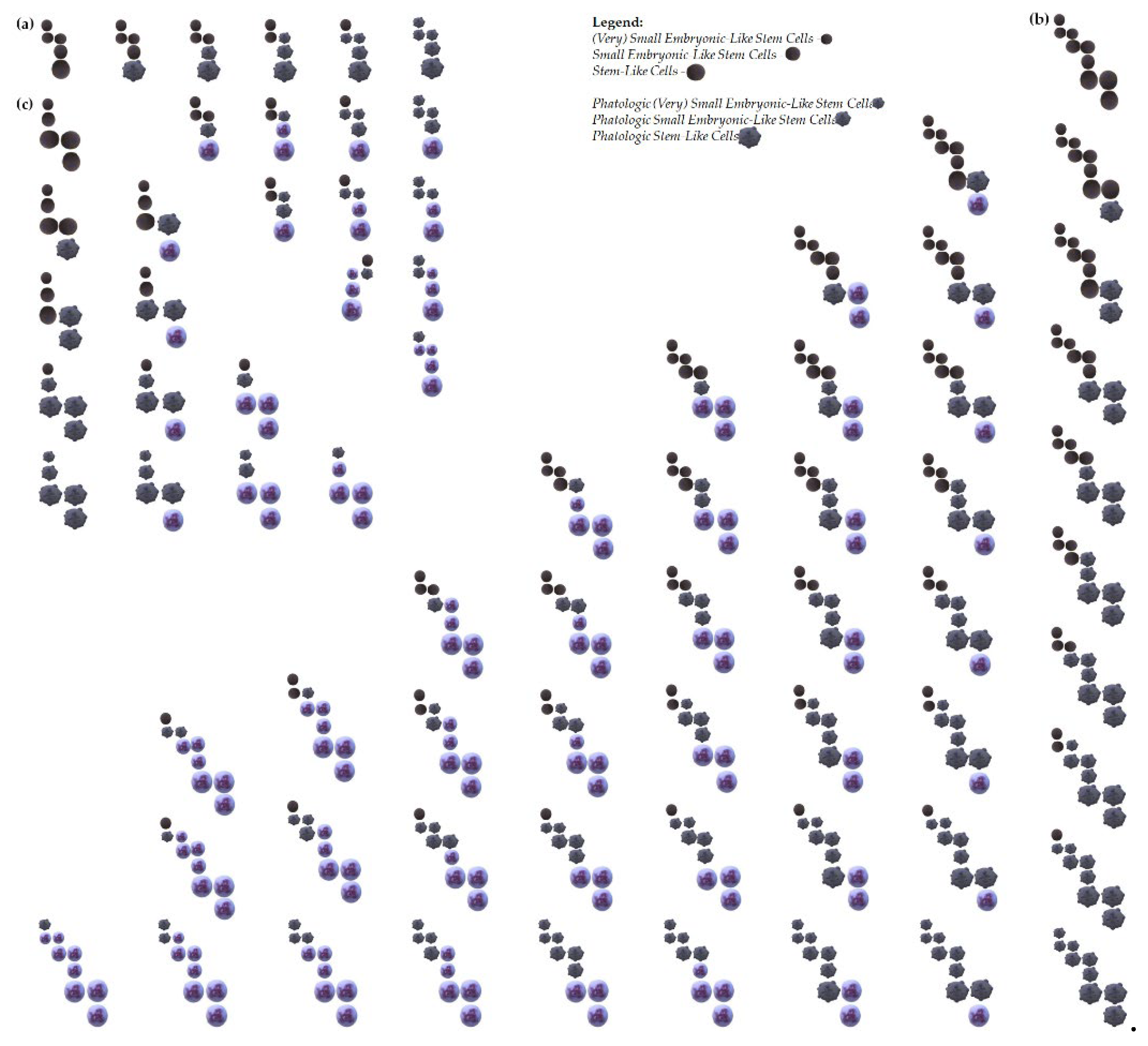
Figure 3.
Proposed examples of acute myeloid leukemia origin from very primitive fusions cell stages. (a) - left panel - Leukemia initiation through the accumulation of changes in pathogenic/pathological cells at different stages in a model where the self-renewing cells are: (V)SELSCs and (V)SLSLCs, SELSCs and SLSLCs as well as SLCs and LSLCs. - right panel - Leukemia initiation at different stages in a model where the self-renewing stages are: (V)SELSCs and (V)SLSLCs, SELSCs and SLSLCs as well as SLCs and LSLCs; (b) - left panel - Leukemia initiation through the accumulation of changes in pathogenic/pathological cells at different stages in a model where the self-renewing and fusion stages are: (V)SELSCs and (V)SLSLCs, SELSCs and SLSLCs as well as SLCs and LSLCs. - right panel – Leukemia initiation at different stages in a model where the self-renewing and fusion stages are: (V)SELSCs and (V)SLSLCs, SELSCs and SLSLCs as well as SLCs and LSLCs; (c) - left panel - Leukemia initiation through the accumulation of changes in pathogenic/pathological cells at different stages in a model where that (V)SELSCs and SELSCs divide asymmetrically, and replication of these cells might occur during LSLCs self-renewing; - right panel - Leukemia initiation at different stages in a model where that (V)SELSCs and SELSCs divide asymmetrically, and replication of these cells might occur during LSLCs self-renewing; In the proposed cell fusion models, we assumed that all cells within a given stage, as well as the first and last cell stages, are capable of fusion - middle stage was not used in this model, however its self-renewal is also probable; It is possible that SELSCs and/or SLSLCs might be a form of (V)SELSCs and/or (V)SLSLCs undergoing self-renewal or asymmetric divisions, and there may be other intermediate stages not included in the presented examples; It is also not excluded that very small or small precursor stages represent a certain form of "dormancy," and their activation into life processes occurs after fusion with a functioning cell.
Figure 3.
Proposed examples of acute myeloid leukemia origin from very primitive fusions cell stages. (a) - left panel - Leukemia initiation through the accumulation of changes in pathogenic/pathological cells at different stages in a model where the self-renewing cells are: (V)SELSCs and (V)SLSLCs, SELSCs and SLSLCs as well as SLCs and LSLCs. - right panel - Leukemia initiation at different stages in a model where the self-renewing stages are: (V)SELSCs and (V)SLSLCs, SELSCs and SLSLCs as well as SLCs and LSLCs; (b) - left panel - Leukemia initiation through the accumulation of changes in pathogenic/pathological cells at different stages in a model where the self-renewing and fusion stages are: (V)SELSCs and (V)SLSLCs, SELSCs and SLSLCs as well as SLCs and LSLCs. - right panel – Leukemia initiation at different stages in a model where the self-renewing and fusion stages are: (V)SELSCs and (V)SLSLCs, SELSCs and SLSLCs as well as SLCs and LSLCs; (c) - left panel - Leukemia initiation through the accumulation of changes in pathogenic/pathological cells at different stages in a model where that (V)SELSCs and SELSCs divide asymmetrically, and replication of these cells might occur during LSLCs self-renewing; - right panel - Leukemia initiation at different stages in a model where that (V)SELSCs and SELSCs divide asymmetrically, and replication of these cells might occur during LSLCs self-renewing; In the proposed cell fusion models, we assumed that all cells within a given stage, as well as the first and last cell stages, are capable of fusion - middle stage was not used in this model, however its self-renewal is also probable; It is possible that SELSCs and/or SLSLCs might be a form of (V)SELSCs and/or (V)SLSLCs undergoing self-renewal or asymmetric divisions, and there may be other intermediate stages not included in the presented examples; It is also not excluded that very small or small precursor stages represent a certain form of "dormancy," and their activation into life processes occurs after fusion with a functioning cell.
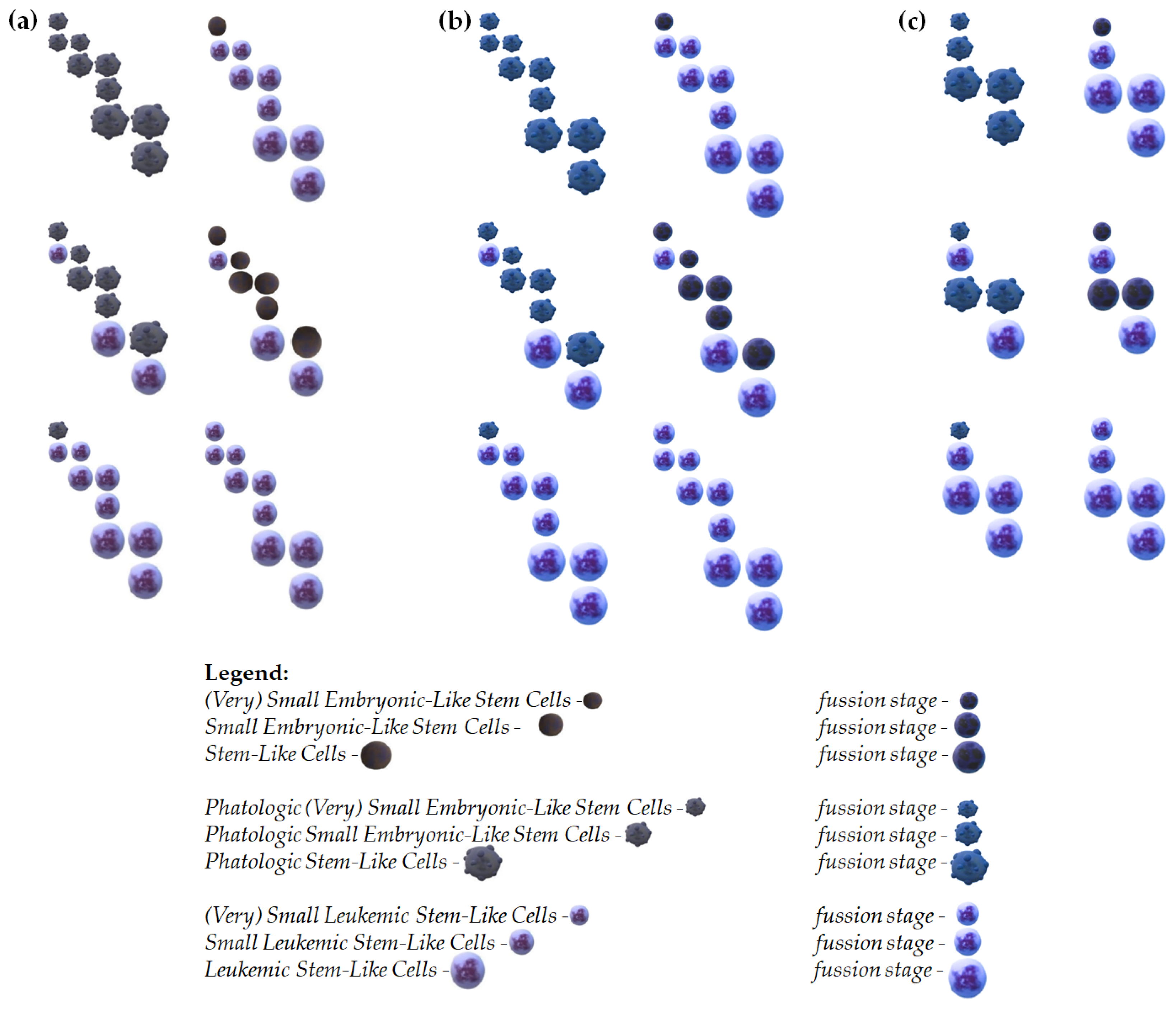
Table 2.
Cytological characteristics and comparison of the very primitive cell stages with the cellular debris. Aberrative: VSELSCs - Very Small Embryonic-Like Stem Cells, (V)SLSLCs - (Very) Small Leukemic Stem-Like Cells, LSLCs - Leukemic Stem-Like Cells, ABs - Apoptotic Bodies, EVs - Extracellular Vesicles. Information about (V)SLSLCs was sourced from [
21], while data on LSLCs was obtained from [
18,
22,
23].
Table 2.
Cytological characteristics and comparison of the very primitive cell stages with the cellular debris. Aberrative: VSELSCs - Very Small Embryonic-Like Stem Cells, (V)SLSLCs - (Very) Small Leukemic Stem-Like Cells, LSLCs - Leukemic Stem-Like Cells, ABs - Apoptotic Bodies, EVs - Extracellular Vesicles. Information about (V)SLSLCs was sourced from [
21], while data on LSLCs was obtained from [
18,
22,
23].
Type of Event |
Cytological Parameter |
VSELSCs |
(V)SLSLCs |
LSLCs |
ABs |
EVs |
Median size (diameter) |
around 5-7 µm |
around 5-7 µm |
around 9-12 µm |
1-5 µm |
0.1-1 µm |
Membrane integrity |
preserved |
preserved |
preserved |
unusual |
unusual |
Specific cell surface markers |
yes |
yes |
yes |
unusual |
unusual |
Cytoplasm |
yes |
yes |
yes |
unusual |
yes |
DNA |
yes |
yes |
yes |
yes |
unusual |
Cell fusion/absorption |
yes |
yes |
yes |
yes |
yes |
Self-renewal |
yes |
yes |
yes |
no |
no |
|
Disclaimer/Publisher’s Note: The statements, opinions and data contained in all publications are solely those of the individual author(s) and contributor(s) and not of MDPI and/or the editor(s). MDPI and/or the editor(s) disclaim responsibility for any injury to people or property resulting from any ideas, methods, instructions or products referred to in the content. |
© 2024 by the authors. Licensee MDPI, Basel, Switzerland. This article is an open access article distributed under the terms and conditions of the Creative Commons Attribution (CC BY) license (http://creativecommons.org/licenses/by/4.0/).